Protective Immunity to SARS-CoV-2
The FLARE Four
- Evidence suggests that humans will develop protective immunity after infection with SARS-CoV-2, although immunity may wane with time
- An effective SARS-CoV-2 vaccine will not be available for the first wave of the pandemic, but will be critical if SARS-CoV-2 establishes itself within the human population
- There are six distinct vaccine platforms being pursued, some with the potential for rapid expansion and large-scale production
- Investment in vaccine development infrastructure is needed to allow for more effective vaccines in response to novel pathogens
Many people are asking...how do we develop a SARS-CoV-2 vaccine?
Subscribe to the latest updates from FLARE Advances in Motion
Introduction
Limited or absent pre-existing immunity to SARS-CoV-2 antigens and the high transmissibility of the virus are the central reasons for the severity of the outbreak of COVID-19. In this FLARE, we will discuss protective immunity to respiratory viruses such as SARS-CoV-2, the challenges of generating an effective respiratory virus vaccine, the SARS-CoV-2 vaccine pipeline, and the investments needed to improve rapid development of vaccines in response to novel pathogens.
Humans Likely Possess Protective Immunity to SARS-CoV-2 After Infection
During the COVID-19 pandemic, there have been reports in the literature and media of individuals recovering from COVID-19 who had negative SARS-CoV-2 PCR results followed by recurrent positive results (Lan et al. 2020). Popular media outlets have subsequently raised the concern that individuals can be rapidly re-infected with SARS-CoV-2 and some health officials have speculated that viral “re-activation” is also possible (“Can you be re-infected after recovering from coronavirus?” Time Magazine 2020). However, notably, in the published reports, the individuals who tested positive for SARS-CoV-2 by PCR after previously testing negative remained asymptomatic (Lan et al. 2020). Consequently, rather than representing re-infection or re-activation, these cases almost certainly represent initial false negative test results during ongoing viral RNA shedding, with subsequent true positive test results.
Human challenge experiments with non-SARS coronaviruses, which generally cause mild upper respiratory tract infections, have demonstrated that humans do develop protective immunity against experimental viral re-challenge (Reed 1984; Huang et al., n.d.). In addition, studies of individuals who recovered from SARS and MERS demonstrate the development of antigen-specific immune memory (W. Liu et al. 2006; Cao et al. 2007; Mo et al. 2006; Memish et al. 2020; Tang et al. 2011; Wu et al. 2007). While we cannot definitively infer that the presence of viral-specific antibodies and/or T cells provide immunity upon re-exposure, the presence of immune memory after SARS and MERS is encouraging and suggests protection compared to naive individuals. Lastly, a small, preliminary pre-print study using a rhesus macaque model of SARS-CoV-2 infection suggested that macaques re-challenged with SARS-CoV-2 28 days after a primary infection could not be infected (Bao et al. 2020). In sum, while we do not yet have a definitive study demonstrating that humans will be protected from rapid SARS-CoV-2 re-infection, there is no evidence to suggest that SARS-CoV-2 infection will preclude the development of protective immunity.
How Long Does Infection-Induced Immunity Last?
Although the mechanisms are incompletely understood, the duration of antigen-specific immune memory is variable (Slifka and Amanna 2019). Human challenge experiments with non-SARS coronaviruses suggest that protective immunity may wane after one year (Callow et al. 1990; Huang et al., n.d.). Studies involving survivors of SARS and MERS indicate the viral-specific antibodies wane significantly after two years with antigen-specific memory B cells being undetectable after six years (Cao et al. 2007; Memish et al. 2020; W. Liu et al. 2006; Tang et al. 2011; Mo et al. 2006; Wu et al. 2007).
Interestingly, antigen-specific T cells seem to be more durable (Tang et al. 2011; Zhao et al. 2017). In both SARS and MERS, individuals who suffered more severe primary infection (typically defined by need for ICU admission or mechanical ventilation) seemed to exhibit more long-lasting, antigen-specific immune memory (Memish et al. 2020; Tang et al. 2011; Zhao et al. 2017).
Thus, while it seems likely that individuals who recover from COVID-19 will acquire protective immunity, the duration of such protection remains unknown and may be related to the severity of illness. Consequently, if SARS-CoV-2 establishes itself in the human population and protective immunity wanes over time, the development of an effective vaccine will be needed to protect both naïve individuals as well as survivors of COVID-19 with waning immunity.
What About Vaccine-Induced Immunity?
Vaccine-induced immune memory may wane faster than immunity from natural infection. For example, as shown in the figure, influenza vaccine effectiveness has been shown to wane quickly with decreased protection at the end of the influenza season (Castilla et al. 2013; Kissling et al. 2016; Krammer, García-Sastre, and Palese 2018). Prime-boost strategies (e.g. serial immunizations with the same antigen or distinct antigens) can be used to enhance the magnitude and duration of immune memory, but additional insight into the signals that yield long-lasting protective immunity will be necessary to achieve more durable protective immunity (Luke and Subbarao 2014).
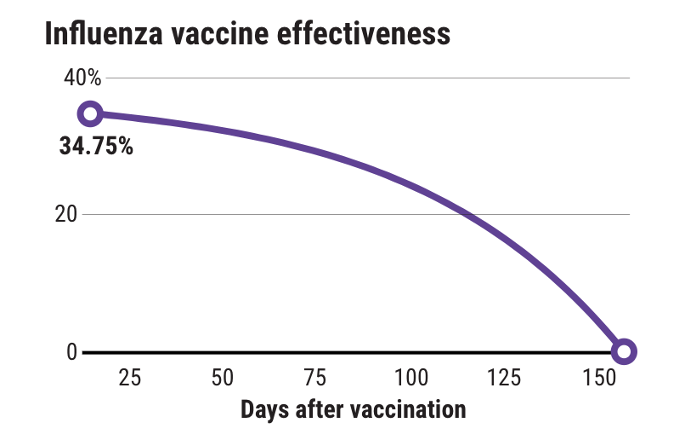
Figure 1
Influenza vaccine effectiveness declines over time (“How Long Do Vaccines Last? The Surprising Answers May Help Protect People Longer” 2019).
Vaccines also seem to induce more limited protective immunity in older individuals, due to poorly-defined, age-associated changes in the immune system termed “immunosenescence” (Boraschi and Italiani 2014). In the context of influenza, high dose vaccination strategies in the elderly have shown greater immunogenicity and protection (Wilkinson et al. 2017). Such biology may become particularly important for a SARS-CoV-2 vaccine given the consistent data that older individuals are at increased risk for severe COVID-19 (Ruan et al. 2020; Guan et al. 2020; Grasselli et al. 2020).
Are There Approaches to Increasing Effectiveness of Vaccine-Induced Immunity for Respiratory Viruses?
Before discussing the platforms for developing a SARS-CoV-2 vaccine, let us review the challenges to producing a vaccine that recapitulates protective immunity achieved from natural infection. Studies from both mouse models and humans have demonstrated that respiratory viral infection induces unique populations of lung-resident memory T and B cells that serve as a frontline defense and enhance protective immunity upon viral re-challenge (Allie et al. 2019; Pizzolla and Wakim 2019). Lung-resident memory T cells can also provide protection against distinct viral serotypes by recognizing more conserved internal viral antigens (Pizzolla and Wakim 2019).
Consequently, there is interest in being able to induce lung-resident memory to enhance host protection. However, we currently do not have a vaccine approach to safely and effectively induce lung-resident memory to combat respiratory viruses. In the case of influenza, for example, inactive influenza vaccines are generally administered via the intramuscular route and primarily induce a neutralizing antibody response to viral surface proteins, providing protection in a serotype-specific manner (Krammer 2019). Intranasal live attenuated influenza vaccines (LAIVs) attempt to induce mucosal immune memory (Singanayagam et al. 2018). However, LAIVs have shown variable efficacy for complex reasons (Pebody, McMenamin, and Nohynek 2018).
Which Vaccination Strategies Are Being Pursued for SARS-CoV-2 and What Is the Time Frame?
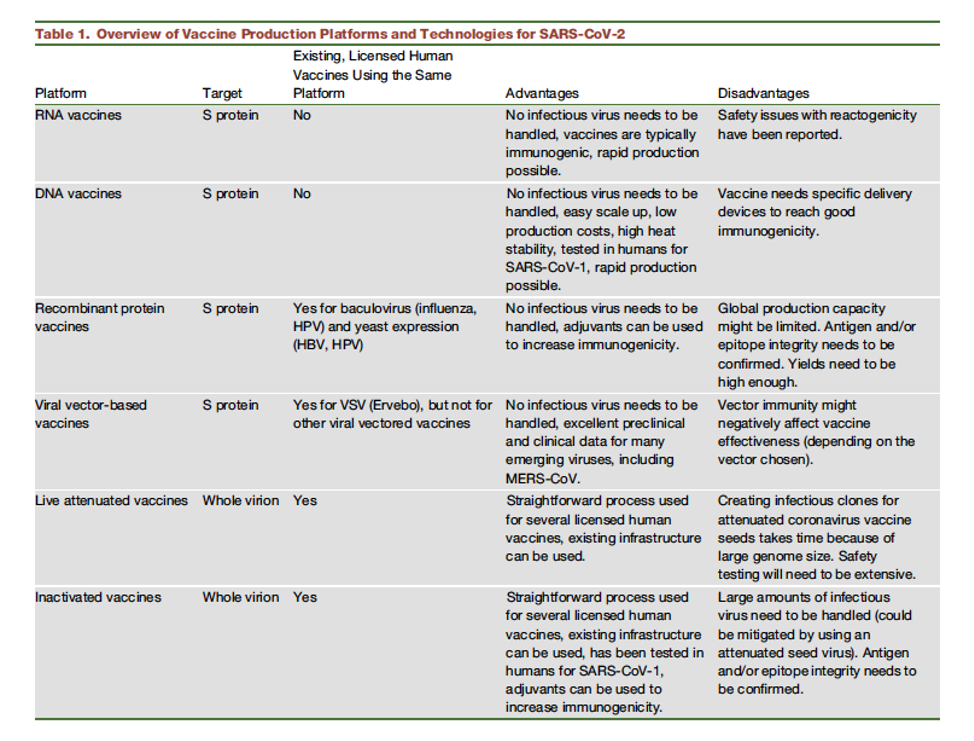
Table 1
Vaccine strategies for SARS-CoV-2 (Amanat and Krammer 2020).
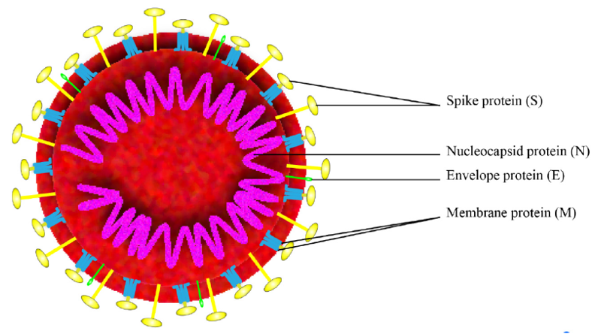
Figure 2
Schematic of the overall structure of SARS-CoV-2 (Zhang et al. 2020).
As described in the table above, six distinct vaccination strategies are being used to develop a vaccine to protect against SARS-CoV-2 (Amanat and Krammer 2020). These strategies focus on the Spike protein, which the virus uses for cell entry, or the whole virion (see figure for reference). Unfortunately, despite the rapid identification and sequencing of SARS-CoV-2, developing a novel vaccine that is effective, safe, and for which mass production is feasible, takes considerable time.
DNA and RNA Vaccines
DNA and RNA-based designs have generated a lot of interest because of their suitability for integration into generic manufacturing platforms (M. A. Liu 2019). Both of these vaccine approaches work by leading to translation of target antigen after cellular uptake of exogenous nucleic acid. The ease and speed of making genetic constructs means that such platforms offer the potential for rapidly designing, constructing, and manufacturing vaccines against epidemic or emerging diseases such as SARS-CoV-2 once the genetic sequence is known.
Vaccines composed of RNA offer some advantages over DNA-based approaches. mRNA is non-infectious and non-integrating (meaning it does not integrate into the host genome - a key concern surrounding DNA vaccines) (Pardi et al. 2018). The basic approach to an mRNA vaccine is as follows. An mRNA molecule that resembles a fully processed eukaryotic messenger RNA and contains an open reading frame that encodes a viral protein of interest is created in vitro. Naked mRNA is quickly degraded in vivo, so the vaccine mRNA is incorporated into some type of delivery system that facilitates cellular uptake, such as lipid nanoparticles. Once intracellular, the mRNA is translated into an antigenic protein that induces an immune response.
An RNA vaccine that was co-developed by Moderna and the Vaccine Research Center at the National Institutes of Health is the furthest along in development of any candidate vaccine. There is an ongoing phase I clinical trial (ClinicalTrials.gov: NCT04283461) involving mRNA encoding SARS-CoV-2 Spike protein antigen encapsulated in lipid nanoparticles. However, no mRNA vaccine for any pathogen has yet reached the market, so this approach faces additional hurdles before safety and efficacy are established.
DNA Vaccines
Wolff et al. first demonstrated that plasmid DNA injected directly into mouse muscle could result in expression of the encoded protein (Wolff et al. 1990). In the setting of SARS-CoV-2, DNA vaccines typically involve encoding the Spike protein. A potential hurdle to DNA-based vaccines is the risk of incorporation of the exogenous DNA into the host cell genome and subsequent induction of mutations (M. A. Liu 2019). Inovio Pharmaceuticals has developed a DNA vaccine candidate, which has also begun enrolling in a phase I trial (ClinicalTrials.gov: NCT04336410). Additional DNA-based vaccines are also under development.
Recombinant Protein-Based Vaccines
Recombinant protein-based techniques typically focus on synthesis of the viral Spike protein or its subunits. Potential concerns include limited immunogenicity (though adjuvants can be added to enhance the immune response), and more challenging production, particularly on the massive scale that would be needed to match global demand.
Viral Vector-Based Techniques
A viral vector-based approach is one in which a distinct virus is engineered to express the SARS-CoV-2 Spike protein. This approach has the advantage of the strong immunogenicity of live attenuated vaccines with safety of subunit vaccines, but immunity to the vector itself (rather than the Spike protein) may limit effectiveness.
Live Attenuated and Inactivated Viral Vaccines
Live attenuated and inactivated viral vaccines are technically straightforward and already used for several licensed vaccines. However, generating a live attenuated seed SARS-CoV-2 will take time. Furthermore, production of an inactivated vaccine would require handling a large amount of infectious SARS-CoV-2.
While multiple approaches are being pursued, it is estimated that at least 12-18 months will be required before a vaccine is available. Consequently, a SARS-CoV-2 vaccine will not be available during the first wave of the pandemic. However, as outlined above, if the virus becomes an established human pathogen, an effective vaccine will be critical.
How Can We Be More Prepared in the Future?
For the reader interested in additional details of the current pipelines for a SARS-CoV-2 vaccine, we recommend the excellent perspective piece by Fatima Amanat and Florian Krammer (Amanat and Krammer 2020). In this article, Amanat and Krammer also articulate the importance of investing in infrastructure to allow for more rapid development of vaccines in response to emerging infectious diseases. Specifically, given that only a few types of virus are likely to cause a global pandemic, it would be feasible to perform surveillance of animal reservoirs in order to identify novel viruses with potential to cause respiratory disease. Viral isolates could be used to produce candidate vaccines and allow for testing in animal models and humans. While the next outbreak may not be caused by the exact same virus as identified by our surveillance procedure, if closely related, sequences could be switched and allow rapid movement to late-stage clinical trials. In addition, building the production capacity that could be quickly activated during an outbreak would allow for shorter time frames to widely distribute the new vaccine and limit the morbidity, mortality, and economic crisis. Such approaches would require significant investment and likely global cooperation, but the current pandemic makes the benefits of such investment clear.
Conclusions
While we do not yet have definitive evidence of protective immunity to SARS-CoV-2, multiple lines of experimental evidence suggest that humans will develop protective immunity and not be susceptible to rapid re-infection. Notably, protective immunity to SARS-CoV-2 may wane with time, although the duration is unknown and may be related, in part, to the severity of COVID-19. While a SARS-CoV-2 vaccine will not be available for the first wave of the COVID-19 pandemic, multiple distinct approaches are currently being pursued to develop an effective vaccine.
References:
- Allie, S. Rameeza, John E. Bradley, Uma Mudunuru, Michael D. Schultz, Beth A. Graf, Frances E. Lund, and Troy D. Randall. 2019. “The Establishment of Resident Memory B Cells in the Lung Requires Local Antigen Encounter.” Nature Immunology 20 (1): 97–108.
- Amanat, Fatima, and Florian Krammer. 2020. “SARS-CoV-2 Vaccines: Status Report.” Immunity 52 (4): 583–89.
- Bao, Linlin, Wei Deng, Hong Gao, Chong Xiao, Jiayi Liu, Jing Xue, Qi Lv, et al. 2020. “Reinfection Could Not Occur in SARS-CoV-2 Infected Rhesus Macaques.” bioRxiv. https://doi.org/10.1101/2020.03.13.990226.
- Boraschi, Diana, and Paola Italiani. 2014. “Immunosenescence and Vaccine Failure in the Elderly: Strategies for Improving Response.” Immunology Letters 162 (1 Pt B): 346–53.
- Callow, K. A., H. F. Parry, M. Sergeant, and D. A. J. Tyrrell. 1990. “The Time Course of the Immune Response to Experimental Coronavirus Infection of Man.” Epidemiology and Infection. https://doi.org/10.1017/s0950268800048019.
- Cao, Wu-Chun, Wei Liu, Pan-He Zhang, Fang Zhang, and Jan H. Richardus. 2007. “Disappearance of Antibodies to SARS-Associated Coronavirus after Recovery.” The New England Journal of Medicine 357 (11): 1162–63.
- Castilla, J., I. Martínez-Baz, V. Martínez-Artola, G. Reina, F. Pozo, M. García Cenoz, M. Guevara, et al. 2013. “Decline in Influenza Vaccine Effectiveness with Time after Vaccination, Navarre, Spain, Season 2011/12.” Eurosurveillance. https://doi.org/10.2807/ese.18.05.20388-en.
- Grasselli, Giacomo, Alberto Zangrillo, Alberto Zanella, Massimo Antonelli, Luca Cabrini, Antonio Castelli, Danilo Cereda, et al. 2020. “Baseline Characteristics and Outcomes of 1591 Patients Infected With SARS-CoV-2 Admitted to ICUs of the Lombardy Region, Italy.” JAMA: The Journal of the American Medical Association, April. https://doi.org/10.1001/jama.2020.5394.
- Guan, Wei-Jie, Zheng-Yi Ni, Yu Hu, Wen-Hua Liang, Chun-Quan Ou, Jian-Xing He, Lei Liu, et al. 2020. “Clinical Characteristics of Coronavirus Disease 2019 in China.” The New England Journal of Medicine, February. https://doi.org/10.1056/NEJMoa2002032.
- “How Long Do Vaccines Last? The Surprising Answers May Help Protect People Longer.” 2019. Science | AAAS. April 18, 2019. https://www.sciencemag.org/news/2019/04/how-long-do-vaccines-last-surprising-answers-may-help-protect-people-longer.
- Huang, Angkana T., Bernardo Garcia-Carreras, Matt D. T. Hitchings, Bingyi Yang, Leah Katzelnick, Susan M. Rattigan, Brooke Borgert, et al. n.d. “A Systematic Review of Antibody Mediated Immunity to Coronaviruses: Antibody Kinetics, Correlates of Protection, and Association of Antibody Responses with Severity of Disease.” https://doi.org/10.1101/2020.04.14.20065771.
- Kissling, Esther, Baltazar Nunes, Chris Robertson, Marta Valenciano, Annicka Reuss, Amparo Larrauri, Jean Marie Cohen, et al. 2016. “I-MOVE Multicentre Case–control Study 2010/11 to 2014/15: Is There within-Season Waning of Influenza Type/subtype Vaccine Effectiveness with Increasing Time since Vaccination?” Eurosurveillance. https://doi.org/10.2807/1560-7917.es.2016.21.16.30201.
- Krammer, Florian. 2019. “The Human Antibody Response to Influenza A Virus Infection and Vaccination.” Nature Reviews. Immunology 19 (6): 383–97.
- Krammer, Florian, Adolfo García-Sastre, and Peter Palese. 2018. “Is It Possible to Develop a ‘Universal’ Influenza Virus Vaccine?” Cold Spring Harbor Perspectives in Biology. https://doi.org/10.1101/cshperspect.a028845.
- Lan, Lan, Dan Xu, Guangming Ye, Chen Xia, Shaokang Wang, Yirong Li, and Haibo Xu. 2020. “Positive RT-PCR Test Results in Patients Recovered From COVID-19.” JAMA: The Journal of the American Medical Association, February. https://doi.org/10.1001/jama.2020.2783.
- Liu, Margaret A. 2019. “A Comparison of Plasmid DNA and mRNA as Vaccine Technologies.” Vaccines 7 (2). https://doi.org/10.3390/vaccines7020037.
- Liu, Wei, Arnaud Fontanet, Pan-he Zhang, Lin Zhan, Zhong-tao Xin, Laurence Baril, Fang Tang, Hui Lv, and Wu-chun Cao. 2006. “Two-Year Prospective Study of the Humoral Immune Response of Patients with Severe Acute Respiratory Syndrome.” The Journal of Infectious Diseases. https://doi.org/10.1086/500469.
- Luke, Catherine J., and Kanta Subbarao. 2014. “Improving Pandemic H5N1 Influenza Vaccines by Combining Different Vaccine Platforms.” Expert Review of Vaccines 13 (7): 873–83.
- Memish, Ziad A., Stanley Perlman, Maria D. Van Kerkhove, and Alimuddin Zumla. 2020. “Middle East Respiratory Syndrome.” The Lancet. https://doi.org/10.1016/s0140-6736(19)33221-0.
- Mo, Hongying, Guangqiao Zeng, Xiaolan Ren, Hui Li, Changwen Ke, Yaxia Tan, Chaoda Cai, et al. 2006. “Longitudinal Profile of Antibodies against SARS-Coronavirus in SARS Patients and Their Clinical Significance.” Respirology 11 (1): 49–53.
- Pardi, Norbert, Michael J. Hogan, Frederick W. Porter, and Drew Weissman. 2018. “mRNA Vaccines - a New Era in Vaccinology.” Nature Reviews. Drug Discovery 17 (4): 261–79.
- Pebody, Richard, Jim McMenamin, and Hanna Nohynek. 2018. “Live Attenuated Influenza Vaccine (LAIV): Recent Effectiveness Results from the USA and Implications for LAIV Programmes Elsewhere.” Archives of Disease in Childhood 103 (1): 101–5.
- Pizzolla, Angela, and Linda M. Wakim. 2019. “Memory T Cell Dynamics in the Lung during Influenza Virus Infection.” Journal of Immunology 202 (2): 374–81.
- Reed, Sylvia E. 1984. “The Behaviour of Recent Isolates of Human Respiratory Coronavirus in Vitro and in Volunteers: Evidence of Heterogeneity among 229E-Related Strains.” Journal of Medical Virology 13 (2): 179–92.
- Ruan, Qiurong, Kun Yang, Wenxia Wang, Lingyu Jiang, and Jianxin Song. 2020. “Clinical Predictors of Mortality due to COVID-19 Based on an Analysis of Data of 150 Patients from Wuhan, China.” Intensive Care Medicine, March. https://doi.org/10.1007/s00134-020-05991-x.
- Singanayagam, Anika, Maria Zambon, Ajit Lalvani, and Wendy Barclay. 2018. “Urgent Challenges in Implementing Live Attenuated Influenza Vaccine.” The Lancet Infectious Diseases 18 (1): e25–32.
- Slifka, Mark K., and Ian J. Amanna. 2019. “Role of Multivalency and Antigenic Threshold in Generating Protective Antibody Responses.” Frontiers in Immunology 10 (May): 956.
- Tang, Fang, Yan Quan, Zhong-Tao Xin, Jens Wrammert, Mai-Juan Ma, Hui Lv, Tian-Bao Wang, et al. 2011. “Lack of Peripheral Memory B Cell Responses in Recovered Patients with Severe Acute Respiratory Syndrome: A Six-Year Follow-up Study.” Journal of Immunology 186 (12): 7264–68.
- Wilkinson, Krista, Yichun Wei, Andrea Szwajcer, Rasheda Rabbani, Ryan Zarychanski, Ahmed M. Abou-Setta, and Salaheddin M. Mahmud. 2017. “Efficacy and Safety of High-Dose Influenza Vaccine in Elderly Adults: A Systematic Review and Meta-Analysis.” Vaccine 35 (21): 2775–80.
- Wolff, J. A., R. W. Malone, P. Williams, W. Chong, G. Acsadi, A. Jani, and P. L. Felgner. 1990. “Direct Gene Transfer into Mouse Muscle in Vivo.” Science 247 (4949 Pt 1): 1465–68.
- Wu, Li-Ping, Nai-Chang Wang, Yi-Hua Chang, Xiang-Yi Tian, Dan-Yu Na, Li-Yuan Zhang, Lei Zheng, Tao Lan, Lin-Fa Wang, and Guo-Dong Liang. 2007. “Duration of Antibody Responses after Severe Acute Respiratory Syndrome.” Emerging Infectious Diseases. https://doi.org/10.3201/eid1310.070576.
- Zhang, Jinyong, Hao Zeng, Jiang Gu, Haibo Li, Lixin Zheng, and Quanming Zou. 2020. “Progress and Prospects on Vaccine Development against SARS-CoV-2.” Vaccines 8 (2). https://doi.org/10.3390/vaccines8020153.
- Zhao, Jingxian, Abeer N. Alshukairi, Salim A. Baharoon, Waleed A. Ahmed, Ahmad A. Bokhari, Atef M. Nehdi, Laila A. Layqah, et al. 2017. “Recovery from the Middle East Respiratory Syndrome Is Associated with Antibody and T-Cell Responses.” Science Immunology 2 (14). https://doi.org/10.1126/sciimmunol.aan5393.
View all FLARE content
Learn more about research in the Division of Pulmonary and Critical Care Medicine