Will COVID-19 cause more IPF?
The FLARE Four
- Many ARDS survivors have evidence of persistent fibrotic lung disease such as radiographic abnormalities and findings of restriction on pulmonary function testing. This has led to concern that the COVID-19 pandemic will result in an increase in the incidence of fibrotic lung disease
- Pre-COVID-19, post-ARDS fibrosis did not appear to behave like progessive interstitial lung diseases such as idiopathic pulmonary fibrosis (IPF)
- COVID-19 and IPF share similar risk factors. It will therefore be difficult to definitively attribute the development of fibrosis in a patient who has survived COVID-19 specifically to COVID-19
- There are no data yet on the incidence or consequences of COVID-19 in patients with pre-existing IPF, though we would expect that they will have worse outcomes
Many people are saying...with high rates of COVID-19 ARDS, we will be seeing more pulmonary fibrosis in the coming years.
Subscribe to the latest updates from FLARE Advances in Motion
Introduction
Fibrotic lung disease has been described as a complication of prolonged respiratory failure from ARDS (Tomashefski 2000). There have therefore been concerns expressed that COVID-19 will lead to large numbers of patients with progressive pulmonary fibrosis (Spagnolo et al. 2020). In tonight’s FLARE, we examine the pathological patterns and clinical presentations of post-ARDS fibrosis and discuss how these data can inform our expectations of long term outcomes in COVID-19 ARDS. We also specifically examine the assertion that COVID-19 will necessarily lead to large numbers of patients with IPF-like illness who would not otherwise have developed the disease, and review the limited data on indications for anti-fibrotic therapies in these patients. Finally, we touch on the limited data describing the outlook for patients with pre-existing fibrosis who develop concurrent COVID-19.
Effects of Post-ARDS Fibrosis on Lung Function
Poor functional status and decreased exercise capacity are well established sequelae in ARDS survivors (Benington et al. 2012). Abnormalities in pulmonary function testing, while initially present, resolve with time in most patients. The Canadian Critical Care Trials Group evaluated 109 survivors of ARDS at multiple time points after ICU discharge (3, 6 and 12 months, 2, 3, 4, and 5 years) (Herridge et al. 2003, 2011). The study showed that, on average, lung volumes and spirometry were normal by 6 months after ICU discharge, but a mild to moderate reduction in DLCO persisted for 12 months. However, the study also found significant functional disability in many patients one year after discharge. As reviewed in a prior FLARE (May 20), most patients had extrapulmonary complications (muscle weakness, fatigue, cognitive impairment) rather than restriction from pulmonary fibrosis (Herridge et al. 2003, 2011). When looking at 5-year outcomes post-ARDS, this same group found that pulmonary function remained stable by 5 years from discharge from the ICU, and by this time, DLCO abnormalities had mostly resolved as well (Herridge et al. 2003, 2011). Continued disability, while present, was primarily attributed to neuromuscular weakness (Orme et al. 2003). It is important to note that this was a relatively young, previously healthy cohort of patients: median age of 44 years, 83% with none or one co-existing medical condition and 83% were working full time when they developed ARDS. These data illustrate that impaired pulmonary function is not the primary cause of post-ICU disability.
However, a minority of patients have persistent restrictive abnormalities on PFTs (Burnham et al. 2013) and many have some residual abnormalities on imaging (Desai et al. Radiology 1999). Both of these findings may be consistent with fibrosis.
Do ARDS Survivors Develop Long Lasting Radiographic Abnormalities?
Yes. 25-85% of post-ARDS patients have residual changes consistent with fibrosis on chest imaging (Sheard, Rao, and Devaraj 2012; Desai et al. 1999). The most commonly documented abnormalities include residual ground glass opacities and reticular markings, which are typically seen in the anterior or non-dependent regions of the lung (Desai et al. 1999). Other studies have shown a more diffuse pattern of reticular and ground glass opacities, with a smaller percent (18%) reported to have exclusively posterior abnormalities (Masclans et al. 2011). It is hypothesized that the regions of ground glass represent fine fibrosis and the abnormalities in the anterior region is the result of barotrauma or over-distention (Sheard, Rao, and Devaraj 2012; Desai et al. 1999) (Figure 1). However, there are not sufficient data from histopathologic specimens to confirm this hypothesis (Sheard, Rao, and Devaraj 2012; Desai et al. 1999).
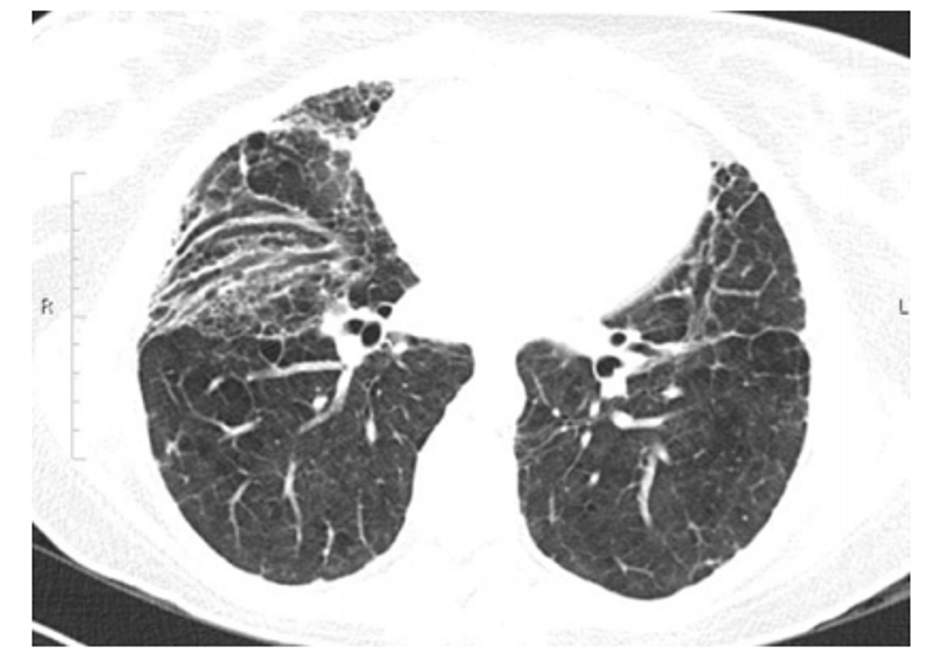
Figure 1
Post-ARDS residual anterior ground glass opacities, reticular markings, and traction bronchiectasis 18 months after the illness (Burnham et al. 2014).
Do Persistent Radiographic Abnormalities Correlate With Impaired Pulmonary Function?
Notwithstanding the results of Herridge et. al., other observational studies of ARDS survivors demonstrated higher rates of pulmonary functional limitations. An observational study in Spain identified 204 patients with ARDS, and out of 93 survivors they followed 38 for post-ARDS lung function and quality of life analysis (Masclans et al. 2011). This cohort was slightly older than the Herridge cohort, with a median age of 53 in the survivor group. In this study, the authors reported higher rates of restrictive lung disease on PFTs (58%) of which 52% was mild restriction.
To the extent that restrictive abnormalities on PFTs may also be due to neuromuscular dysfunction and not parenchymal abnormalities, correlation of imaging abnormalities and PFT abnormalities could be helpful in diagnosing true fibrotic changes. However, there are few studies correlating CT abnormalities (either during acute illness or long-term changes) and long-term pulmonary function. In one notable study, burden of disease on CT scans 14 days into hospitalization for ARDS predicted impairment in pulmonary function 180 days post-illness (Burnham et al. 2013).
Is Post-ARDS Fibrosis Similar to Progressive Fibrotic Lung Diseases Such as IPF?
It is not well understood why some ARDS survivors have complete resolution of their lung disease and others progress to fibrosis. There are some data to suggest increased rates of fibrosis in patients who spend a longer time on the ventilator and had higher PEEP levels, suggesting that more severe and prolonged illness may be a risk factor (Desai et al. 1999). Patients with pulmonary causes of ARDS have also been shown to have higher rates of fibrosis post-ARDS than those with extrapulmonary causes of ARDS (Kim et al. 2004). In the currently available cohorts, including the Canadian Clinical Trials post-ARDS cohort (Herridge et al. 2011), patients had stable impairment in pulmonary function over the 5 year follow-up period despite having restrictive PFTs (Herridge et al. 2011) - demonstrating a lack of progression that is very unlike de novo IPF. There have also not been any studies suggesting that patients with post-ARDS fibrosis develop acute exacerbations like we see in IPF, though admittedly data are limited.
COVID-19 and IPF: Common Risk Factors, Unclear Interaction
A recent review discussed the fact that COVID-19 and IPF share similar risk factors (George, Wells, and Jenkins 2020). The authors noted that the major risk factors for both severe COVID-19 and IPF are older age, male sex, a history of smoking, and comorbidities such as diabetes, ischemic heart disease and hypertension (C. S. King and Nathan 2017). These common risk factors may confound any conclusion about causality between COVID-19 ARDS and subsequently diagnosed IPF. Additionally, since patients at risk for IPF are also at risk for critical COVID-19, it is natural to wonder how patients with IPF (including sub-clinical IPF) will tolerate COVID-19. Although it is tempting to speculate that patients with subclinical interstitial lung disease (ILD) could develop accelerated disease in the context of COVID-19 infection, data are not yet available to confirm this. Additionally, although patients with ILD, including IPF, are known to have poorer prognosis with ARDS (Gannon et al. 2018; Rush et al. 2016) compared to ARDS in non-ILD patients (Bellani et al. 2016), we do not yet have data confirming this to be the case with COVID-19. Lastly, it remains unclear whether anti-fibrotic medications (which IPF patients may be on at the time of COVID-19 diagnosis) will offer any protection, and there may be an insufficient number of patients with IPF on therapy to answer this question.
Are Patients With Severe COVID-19 ARDS Developing Fibrosis?
Although it is too early in this pandemic to identify the incidence of post-COVID-19 ARDS fibrosis, multiple studies of prior global viral respiratory illness outbreaks may offer some insight. In studies of survivors of SARS and MERS, it was found that approximately 30% of survivors developed persistent radiographic abnormalities and 15% developed physiologic restriction. Risk factors for fibrosis were older age and more severe disease requiring ICU admission (Das et al. 2017; Hui et al. 2005). Studies during the COVID-19 outbreak in China have established both persistent radiographic patterns of GGO and consolidation (Shi et al. 2020) and abnormal lung function (Mo et al. 2020) at the time of hospital discharge. However, long term outcome data are needed in order to accurately predict how many COVID-19 ARDS survivors will have persistent and clinically significant abnormal lung function.
Is the Pathologic Pattern of COVID-19 ARDS Similar to Prior Studies of ARDS Patients?
As discussed in a prior FLARE, multiple histologic studies of patients with COVID-19 associated respiratory failure have shown that diffuse alveolar damage, the histologic correlate of severe ARDS, is the predominant histologic pattern observed in COVID-19 (Xu et al. 2020; Schaller et al. 2020; Zhang et al. 2020; Ackermann et al. 2020). A proportion of those patients who progress to the chronic, fibrotic phase of DAD will develop progressive architectural remodeling and interstitial fibrosis. In extreme cases, these changes may be so severe as to resemble (Tomashefski 2000) usual interstitial pneumonitis (UIP), the histologic correlate of idiopathic pulmonary fibrosis (IPF).
Could Anti-fibrotics Prevent Progressive Pulmonary Fibrosis After COVID-19?
A recent opinion piece considered a potential role for studying antifibrotic therapy in COVID-19 (George, Wells, and Jenkins 2020). The authors note that several of the antifibrotic agents currently in use or in clinical development for pulmonary fibrosis have been studied in pre-clinical animal models of acute lung injury. While the bleomycin injury model has long been criticized as a poor model of progressive lung fibrosis because fibrosis invariably resolves (Chua, Gauldie, and Laurent 2005), this may represent an ideal model of fibroproliferative ARDS.
The major outcomes for the two currently approved antifibrotic medications (pirfenidone and nintedanib) medications have been measured over the course of one to five years (T. E. King Jr et al. 2014; Richeldi et al. 2014; Zurkova et al. 2019; Antoniou et al. 2020). However, at least in the case of nintedanib, the benefit was evident by 4-6 weeks. George and colleagues suggest that this might mean antifibrotic therapy attenuates antifibrotic pathways within weeks after administration. Furthermore, both nintedanib and pirfenidone have been tested in fibrotic lung diseases other than IPF, opening up the possibility for secondary uses of these medications. In the INBUILD trial, nintedanib was an effective anti-fibrotic drug across a range of fibrotic lung conditions (Flaherty et al. 2019). Additionally, nintedanib specifically may reduce acute exacerbations of interstitial lung disease (Richeldi et al. 2014, 2016). While the cause of ILD exacerbations is not clear, there is some evidence that exacerbations may be caused by respiratory viruses, such as coronavirus (Wootton et al. 2011). Therefore, it is possible that the same mechanism of these drugs that prevents exacerbations could potentially mitigate the effect of COVID-19 on lung fibrosis. This is, however, an unproven hypothesis.
There are additional drugs in development for pulmonary fibrosis which may also have activity against the SARS-CoV-2 virus (Figure 2). For example, the SARS-CoV-2 spike protein has an integrin binding domain and coronaviruses contain a galectin fold (Li 2015). Mice with genetic deletion of ανβ6 integrins are protected in viral induced lung injury models and also in acute lung injury models with bleomycin (Jolly et al. 2014; Meliopoulos et al. 2016; Jenkins 2006). Galectin-3 knockout mice are protected from pulmonary inflammation after influenza infection in addition to bleomycin induced pulmonary fibrosis (Spackman 2016; Bartolazzi 2018). These are therefore potential targets for IPF, SARS-CoV-2, and potential COVID-induced fibrosis. There are also two recent protein-protein interaction studies that identified mTOR as an anti-SARS-CoV-2 target, suggesting rapamycin as a potential therapeutic option (Gopi Mohan 2019; Gordon et al. 2020). Interestingly, mTOR has also been an emerging target in IPF, as it was observed in a large GWAS study (Spagnolo and Kropski 2020). Lastly, PI3K inhibitors have shown acceptable safety profiles in early studies in IPF (Lukey et al. 2019) (Figure 3).
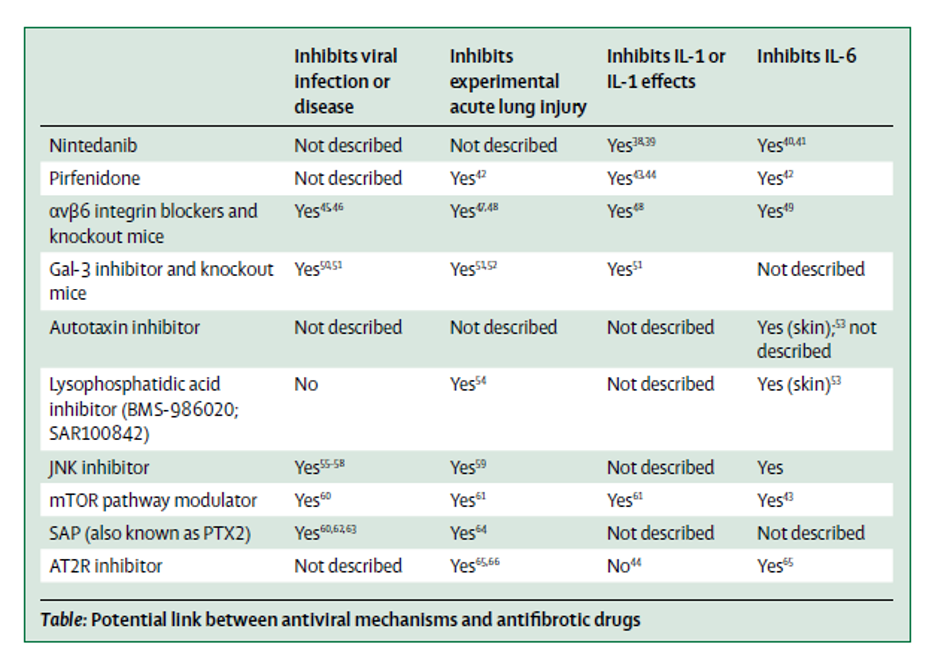
Figure 2
Potential Links between antiviral mechanisms and antifibrotic drugs (George et al. Lancet 2020).
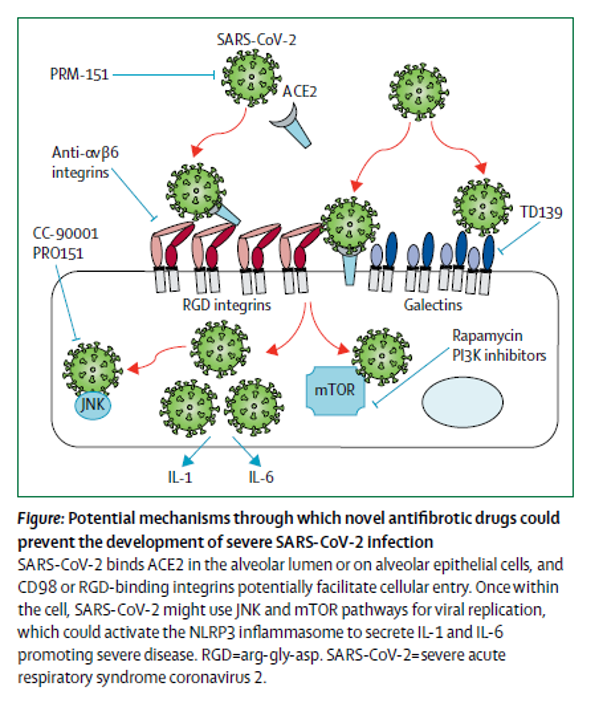
Figure 3
Potential mechanisms through which novel antifibrotic drugs could prevent the development of severe SARS-CoV-2 infection (George et al. Lancet 2020).
Another therapeutic target that has garnered much attention in COVID-19 is IL-6, which is generally considered to be a pro-fibrotic cytokine. However, in animal studies of bleomycin-induced lung injury, there are two spikes of IL-6 after bleomycin injury. Inhibition of the early IL-6 response after injury actually accelerated fibrosis, whereas inhibition blocking the second spike reduced the fibrotic response (Kobayashi et al. 2015). Thus, caution should be used if blocking IL-6 as incorrectly timed IL-6 blockade has the potential to worsen fibrosis.
Conclusions
In summary, previous studies of ARDS have suggested that up to 25% of ARDS survivors will develop physiologic evidence of restrictive lung disease on PFTs 6 months from diagnosis. In addition, patients with pre-existing fibrosis, including sub-clinical fibrosis, may have worse outcomes in the setting of COVID-19, though more data is needed. The numbers of COVID-19 ARDS patients we have seen in the last several months would predict that we are likely going to see increased numbers of patients with fibrotic lung disease as a complication of their ARDS within the next year. Although this fibrotic scarring may lead to some long-term functional limitations, post-ARDS fibrosis does not appear to progress the same way as IPF, for unclear reasons. Given long-term fibrotic sequelae, and the possible association between pre-existing fibrosis and poor outcome, proposals are emerging to study anti-fibrotics in COVID-19 ARDS. However, there are limitations to considering these drugs, including rapidity of action, timing, mode of delivery, and side effect profiles.
References
- Ackermann, Maximilian, Stijn E. Verleden, Mark Kuehnel, Axel Haverich, Tobias Welte, Florian Laenger, Arno Vanstapel, et al. 2020. “Pulmonary Vascular Endothelialitis, Thrombosis, and Angiogenesis in Covid-19.” The New England Journal of Medicine, May. https://doi.org/10.1056/NEJMoa2015432.
- Antoniou, Katerina, Katerina Markopoulou, Argyrios Tzouvelekis, Athina Trachalaki, Eirini Vasarmidi, Jiannis Organtzis, Vasilios Tzilas, et al. 2020. “Efficacy and Safety of Nintedanib in a Greek Multicentre Idiopathic Pulmonary Fibrosis Registry: A Retrospective, Observational, Cohort Study.” ERJ Open Research 6 (1). https://doi.org/10.1183/23120541.00172-2019.
- Bartolazzi, Armando. 2018. Galectins in Cancer and Translational Medicine. MDPI.
- Bellani, Giacomo, John G. Laffey, Tài Pham, Eddy Fan, Laurent Brochard, Andres Esteban, Luciano Gattinoni, et al. 2016. “Epidemiology, Patterns of Care, and Mortality for Patients With Acute Respiratory Distress Syndrome in Intensive Care Units in 50 Countries.” JAMA: The Journal of the American Medical Association 315 (8): 788–800.
- Benington, Steve, David McWilliams, Jane Eddleston, and Dougal Atkinson. 2012. “Exercise Testing in Survivors of Intensive Care--Is There a Role for Cardiopulmonary Exercise Testing?” Journal of Critical Care 27 (1): 89–94.
- Burnham, Ellen L., Robert C. Hyzy, Robert Paine 3rd, Curtis Coley 2nd, Aine M. Kelly, Leslie E. Quint, David Lynch, William J. Janssen, Marc Moss, and Theodore J. Standiford. 2013. “Chest CT Features Are Associated with Poorer Quality of Life in Acute Lung Injury Survivors.” Critical Care Medicine 41 (2): 445–56.
- Burnham, Ellen L., William J. Janssen, David W. H. Riches, Marc Moss, and Gregory P. Downey. 2014. “The Fibroproliferative Response in Acute Respiratory Distress Syndrome: Mechanisms and Clinical Significance.” The European Respiratory Journal: Official Journal of the European Society for Clinical Respiratory Physiology 43 (1): 276–85.
- Chua, Felix, Jack Gauldie, and Geoffrey J. Laurent. 2005. “Pulmonary Fibrosis.” American Journal of Respiratory Cell and Molecular Biology. https://doi.org/10.1165/rcmb.2005-0062tr.
- Das, Karuna M., Edward Y. Lee, Rajvir Singh, Mushira A. Enani, Khalid Al Dossari, Klaus Van Gorkom, Sven G. Larsson, and Ruth D. Langer. 2017. “Follow-up Chest Radiographic Findings in Patients with MERS-CoV after Recovery.” The Indian Journal of Radiology & Imaging 27 (3): 342–49.
- Desai, S. R., A. U. Wells, M. B. Rubens, T. W. Evans, and D. M. Hansell. 1999. “Acute Respiratory Distress Syndrome: CT Abnormalities at Long-Term Follow-Up.” Radiology 210 (1): 29–35.
- Flaherty, Kevin R., Athol U. Wells, Vincent Cottin, Anand Devaraj, Simon L. F. Walsh, Yoshikazu Inoue, Luca Richeldi, et al. 2019. “Nintedanib in Progressive Fibrosing Interstitial Lung Diseases.” The New England Journal of Medicine 381 (18): 1718–27.
- Gannon, Whitney D., David J. Lederer, Mauer Biscotti, Azka Javaid, Nina M. Patel, Daniel Brodie, Matthew Bacchetta, and Matthew R. Baldwin. 2018. “Outcomes and Mortality Prediction Model of Critically Ill Adults With Acute Respiratory Failure and Interstitial Lung Disease.” Chest. https://doi.org/10.1016/j.chest.2018.01.006.
- George, Peter M., Athol U. Wells, and R. Gisli Jenkins. 2020. “Pulmonary Fibrosis and COVID-19: The Potential Role for Antifibrotic Therapy.” The Lancet. Respiratory Medicine, May. https://doi.org/10.1016/S2213-2600(20)30225-3.
- Gopi Mohan, C. 2019. Structural Bioinformatics: Applications in Preclinical Drug Discovery Process. Springer.
- Gordon, David E., Gwendolyn M. Jang, Mehdi Bouhaddou, Jiewei Xu, Kirsten Obernier, Kris M. White, Matthew J. O’Meara, et al. 2020. “A SARS-CoV-2 Protein Interaction Map Reveals Targets for Drug Repurposing.” Nature, April. https://doi.org/10.1038/s41586-020-2286-9.
- Herridge, Margaret S., Angela M. Cheung, Catherine M. Tansey, Andrea Matte-Martyn, Natalia Diaz-Granados, Fatma Al-Saidi, Andrew B. Cooper, et al. 2003. “One-Year Outcomes in Survivors of the Acute Respiratory Distress Syndrome.” The New England Journal of Medicine 348 (8): 683–93.
- Herridge, Margaret S., Catherine M. Tansey, Andrea Matté, George Tomlinson, Natalia Diaz-Granados, Andrew Cooper, Cameron B. Guest, et al. 2011. “Functional Disability 5 Years after Acute Respiratory Distress Syndrome.” The New England Journal of Medicine 364 (14): 1293–1304.
- Hui, D. S., G. M. Joynt, K. T. Wong, C. D. Gomersall, T. S. Li, G. Antonio, F. W. Ko, et al. 2005. “Impact of Severe Acute Respiratory Syndrome (SARS) on Pulmonary Function, Functional Capacity and Quality of Life in a Cohort of Survivors.” Thorax 60 (5): 401–9.
- Jenkins, R. G. 2006. “Ligation of Protease-Activated Receptor 1 Enhances v 6 Integrin-Dependent TGF- Activation and Promotes Acute Lung Injury.” Journal of Clinical Investigation. https://doi.org/10.1172/jci27183.
- Jolly, Lisa, Anastasios Stavrou, Gilles Vanderstoken, Victoria A. Meliopoulos, Anthony Habgood, Amanda L. Tatler, Joanne Porte, et al. 2014. “Influenza Promotes Collagen Deposition via αvβ6 Integrin-Mediated Transforming Growth Factor β Activation.” The Journal of Biological Chemistry 289 (51): 35246–63.
- Kim, Sun Jong, Bum Jin Oh, Jin Sung Lee, Chae-Man Lim, Tae Sun Shim, Sang Do Lee, Woo Sung Kim, Dong Soon Kim, Won Dong Kim, and Younsuck Koh. 2004. “Recovery from Lung Injury in Survivors of Acute Respiratory Distress Syndrome: Difference between Pulmonary and Extrapulmonary Subtypes.” Intensive Care Medicine 30 (10): 1960–63.
- King, Christopher S., and Steven D. Nathan. 2017. “Idiopathic Pulmonary Fibrosis: Effects and Optimal Management of Comorbidities.” The Lancet. Respiratory Medicine 5 (1): 72–84.
- King, Talmadge E., Jr, Williamson Z. Bradford, Socorro Castro-Bernardini, Elizabeth A. Fagan, Ian Glaspole, Marilyn K. Glassberg, Eduard Gorina, et al. 2014. “A Phase 3 Trial of Pirfenidone in Patients with Idiopathic Pulmonary Fibrosis.” The New England Journal of Medicine 370 (22): 2083–92.
- Kobayashi, Takeshi, Kensuke Tanaka, Tetsuo Fujita, Hiroki Umezawa, Hiroyuki Amano, Kento Yoshioka, Yusuke Naito, et al. 2015. “Bidirectional Role of IL-6 Signal in Pathogenesis of Lung Fibrosis.” Respiratory Research. https://doi.org/10.1186/s12931-015-0261-z.
- Li, Fang. 2015. “Receptor Recognition Mechanisms of Coronaviruses: A Decade of Structural Studies.” Journal of Virology. https://doi.org/10.1128/jvi.02615-14.
- Lukey, Pauline T., Stephen A. Harrison, Shuying Yang, Yim Man, Beverley F. Holman, Alaleh Rashidnasab, Gabrielle Azzopardi, et al. 2019. “A Randomised, Placebo-Controlled Study of Omipalisib (PI3K/mTOR) in Idiopathic Pulmonary Fibrosis.” European Respiratory Journal. https://doi.org/10.1183/13993003.01992-2018.
- Masclans, Joan R., Oriol Roca, Xavier Muñoz, Esther Pallisa, Ferran Torres, Jordi Rello, and Ferran Morell. 2011. “Quality of Life, Pulmonary Function, and Tomographic Scan Abnormalities after ARDS.” Chest 139 (6): 1340–46.
- Meliopoulos, Victoria A., Lee-Ann Van de Velde, Nicholas C. Van de Velde, Erik A. Karlsson, Geoff Neale, Peter Vogel, Cliff Guy, et al. 2016. “An Epithelial Integrin Regulates the Amplitude of Protective Lung Interferon Responses against Multiple Respiratory Pathogens.” PLOS Pathogens. https://doi.org/10.1371/journal.ppat.1005804.
- Mo, Xiaoneng, Wenhua Jian, Zhuquan Su, Mu Chen, Hui Peng, Ping Peng, Chunliang Lei, Shiyue Li, Ruchong Chen, and Nanshan Zhong. 2020. “Abnormal Pulmonary Function in COVID-19 Patients at Time of Hospital Discharge.” The European Respiratory Journal: Official Journal of the European Society for Clinical Respiratory Physiology, May. https://doi.org/10.1183/13993003.01217-2020.
- Orme, James, Jr, Joshua S. Romney, Ramona O. Hopkins, Donna Pope, Karen J. Chan, George Thomsen, Robert O. Crapo, and Lindell K. Weaver. 2003. “Pulmonary Function and Health-Related Quality of Life in Survivors of Acute Respiratory Distress Syndrome.” American Journal of Respiratory and Critical Care Medicine 167 (5): 690–94.
- Richeldi, Luca, Roland M. du Bois, Ganesh Raghu, Arata Azuma, Kevin K. Brown, Ulrich Costabel, Vincent Cottin, et al. 2014. “Efficacy and Safety of Nintedanib in Idiopathic Pulmonary Fibrosis.” The New England Journal of Medicine 370 (22): 2071–82.
- Richeldi, Luca, Vincent Cottin, Roland M. du Bois, Moisés Selman, Toshio Kimura, Zelie Bailes, Rozsa Schlenker-Herceg, Susanne Stowasser, and Kevin K. Brown. 2016. “Nintedanib in Patients with Idiopathic Pulmonary Fibrosis: Combined Evidence from the TOMORROW and INPULSIS(®) Trials.” Respiratory Medicine 113 (April): 74–79.
- Rush, Barret, Katie Wiskar, Landon Berger, and Donald Griesdale. 2016. “The Use of Mechanical Ventilation in Patients with Idiopathic Pulmonary Fibrosis in the United States: A Nationwide Retrospective Cohort Analysis.” Respiratory Medicine. https://doi.org/10.1016/j.rmed.2015.12.005.
- Schaller, Tina, Klaus Hirschbühl, Katrin Burkhardt, Georg Braun, Martin Trepel, Bruno Märkl, and Rainer Claus. 2020. “Postmortem Examination of Patients With COVID-19.” JAMA: The Journal of the American Medical Association, May. https://doi.org/10.1001/jama.2020.8907.
- Sheard, Sarah, Praveen Rao, and Anand Devaraj. 2012. “Imaging of Acute Respiratory Distress Syndrome.” Respiratory Care 57 (4): 607–12.
- Shi, Heshui, Xiaoyu Han, Nanchuan Jiang, Yukun Cao, Osamah Alwalid, Jin Gu, Yanqing Fan, and Chuansheng Zheng. 2020. “Radiological Findings from 81 Patients with COVID-19 Pneumonia in Wuhan, China: A Descriptive Study.” The Lancet Infectious Diseases 20 (4): 425–34.
- Spackman, Erica. 2016. Animal Influenza Virus. Springer New York.
- Spagnolo, Paolo, Elisabetta Balestro, Stefano Aliberti, Elisabetta Cocconcelli, Davide Biondini, Giovanni Della Casa, Nicola Sverzellati, and Toby M. Maher. 2020. “Pulmonary Fibrosis Secondary to COVID-19: A Call to Arms?” The Lancet. Respiratory Medicine, May. https://doi.org/10.1016/S2213-2600(20)30222-8.
- Spagnolo, Paolo, and Jonathan A. Kropski. 2020. “Genome-Wide Association Studies in Idiopathic Pulmonary Fibrosis: Bridging the Gap between Sequence and Consequence.” American Journal of Respiratory and Critical Care Medicine. https://doi.org/10.1164/rccm.201911-2286ed.
- Tomashefski, J. F., Jr. 2000. “Pulmonary Pathology of Acute Respiratory Distress Syndrome.” Clinics in Chest Medicine 21 (3): 435–66.
- Wootton, Sharon Chao, Dong Soon Kim, Yasuhiro Kondoh, Eunice Chen, Joyce S. Lee, Jin Woo Song, Jin Won Huh, et al. 2011. “Viral Infection in Acute Exacerbation of Idiopathic Pulmonary Fibrosis.” American Journal of Respiratory and Critical Care Medicine. https://doi.org/10.1164/rccm.201010-1752oc.
- Xu, Zhe, Lei Shi, Yijin Wang, Jiyuan Zhang, Lei Huang, Chao Zhang, Shuhong Liu, et al. 2020. “Pathological Findings of COVID-19 Associated with Acute Respiratory Distress Syndrome.” The Lancet. Respiratory Medicine 8 (4): 420–22.
- Zhang, Huilan, Peng Zhou, Yanqiu Wei, Huihui Yue, Yi Wang, Ming Hu, Shu Zhang, et al. 2020. “Histopathologic Changes and SARS-CoV-2 Immunostaining in the Lung of a Patient With COVID-19.” Annals of Internal Medicine 172 (9): 629–32.
- Zurkova, Monika, Eva Kriegova, Vitezslav Kolek, Vladimira Lostakova, Martina Sterclova, Vladimir Bartos, Martina Doubkova, et al. 2019. “Effect of Pirfenidone on Lung Function Decline and Survival: 5-Yr Experience from a Real-Life IPF Cohort from the Czech EMPIRE Registry.” Respiratory Research 20 (1): 16.
View all COVID-19 Updates
Learn more about research in the Division of Pulmonary and Critical Care Medicine