How is SARS-CoV-2 transmitted?
The FLARE Four
- Respiratory particles, produced every time we talk, cough, sneeze, shout, or sing, vary in size, from fine aerosol particles and droplet nuclei (< 5 µm) to large droplets (> 5 µm). Airborne transmission of respiratory pathogens occurs via the dissemination of respirable fine aerosol particles containing organisms that remain infectious over distance and time
- The possible routes of transmission of SARS-CoV-2 are not fully understood, but larger droplets are thought to be the primary mode of transmission. The possibility of so-called opportunistic aerosol spread, wherein special circumstances such as aerosol generating procedures in the hospital facilitate airborne transmission of a normally droplet-transmitted disease, has been proposed (but not proven) for the closely related viruses SARS-CoV and MERS-CoV
- While recent evidence suggests that the SARS-CoV-2 virus may remain aerosolized for hours after aerosol generation, the presence of aerosols should be distinguished from infectious aerosols
- To date, epidemiological analyses of COVID-19 outbreaks and exposure investigations in healthcare settings have not demonstrated airborne transmission of SARS-CoV-2, i.e. there is little evidence that the SARS-CoV-2 virus remains infective over distance and time. However, more data are needed
Many people are asking...is there evidence of airborne transmission of SARS-CoV-2?
Subscribe to the latest updates from FLARE Advances in Motion
Introduction
Knowledge of an emerging pathogen’s mode of transmission is paramount for the development of effective infection control guidelines and public health policies to protect our healthcare facilities and communities. Classically, respiratory pathogens may be divided into those transmitted by the airborne route and by the droplet route. Several factors determine the primary mode of transmission of respiratory infections: respiratory particle size, pathogen-specific factors such as cellular tropism, infectious dose, airflow patterns, and host factors such as mucosal inflammation (Tellier et al. 2019). While we know a great deal about the biophysics of respiratory particles, the pathogen-specific factors that influence the mode of transmission are not easily predicted for a newly discovered disease such as COVID-19. In tonight’s FLARE, we will discuss droplet and airborne modes of transmission and review data from the SARS and COVID-19 pandemics to answer the following question: is there any evidence of airborne transmission of SARS-CoV-2?
Fine Aerosols, Droplets, Droplet vs. Airborne—Let’s Break It Down
Respiratory particles, produced every time we talk, cough, sneeze, shout, or sing, vary in size, from fine aerosols to large droplets. Droplets have been historically defined by CDC as particles > 5 µm in size. Due to their larger size, they are believed to fall to the ground quickly via a ballistic trajectory (Wei and Li 2016). For this reason, diseases transmitted via the droplet route are spread through close person-to-person contact, typically at a distance < 6 feet. Fine particle aerosols and droplet nuclei, on the other hand, are < 5 µm. This distinction matters for two important reasons: 1) fine particle aerosols may potentially remain suspended in the air, and 2) these respirable particles are also just small enough to be inhaled deeper into the respiratory tract whereas larger droplets typically settle in the upper respiratory tract (CDC 2003). These features are what make airborne transmission of infectious organisms through fine aerosols plausible. However, particle size is only one part of the equation. Pathogen-specific and environmental factors likely play an important role in determining whether a pathogen has an airborne transmission pattern (Tellier et al. 2019). The CDC defines airborne transmission as occurring when organisms in respirable particles remain infectious over distance and time, as observed in the spread of tuberculosis. This is known as the classic, long-range airborne transmission route (Figure 1). Classic long-range transmission is established only for a very small number of pathogens - measles and TB being the most often cited (Roy and Milton 2004).
The specific route of infection has implications for infection control and personal protective equipment (PPE). In the case of droplet-based transmission, universal surgical masking can greatly limit transmission by blocking the spread of oral and nasal droplets (Klompas et al. 2020). In the case of true long-range airborne transmission, the ability of infectious droplets to follow airflows requires a mask with a tight seal, and the small size of infectious particles requires enhanced filtering (Tellier et al. 2019). In the current outbreak of SARS-CoV-2, concerns have been raised over the potential airborne transmission, based, in part, on possible instances of airborne transmission of the closely related SARS-CoV and MERS-CoV viruses (Shiu, Leung, and Cowling 2019).
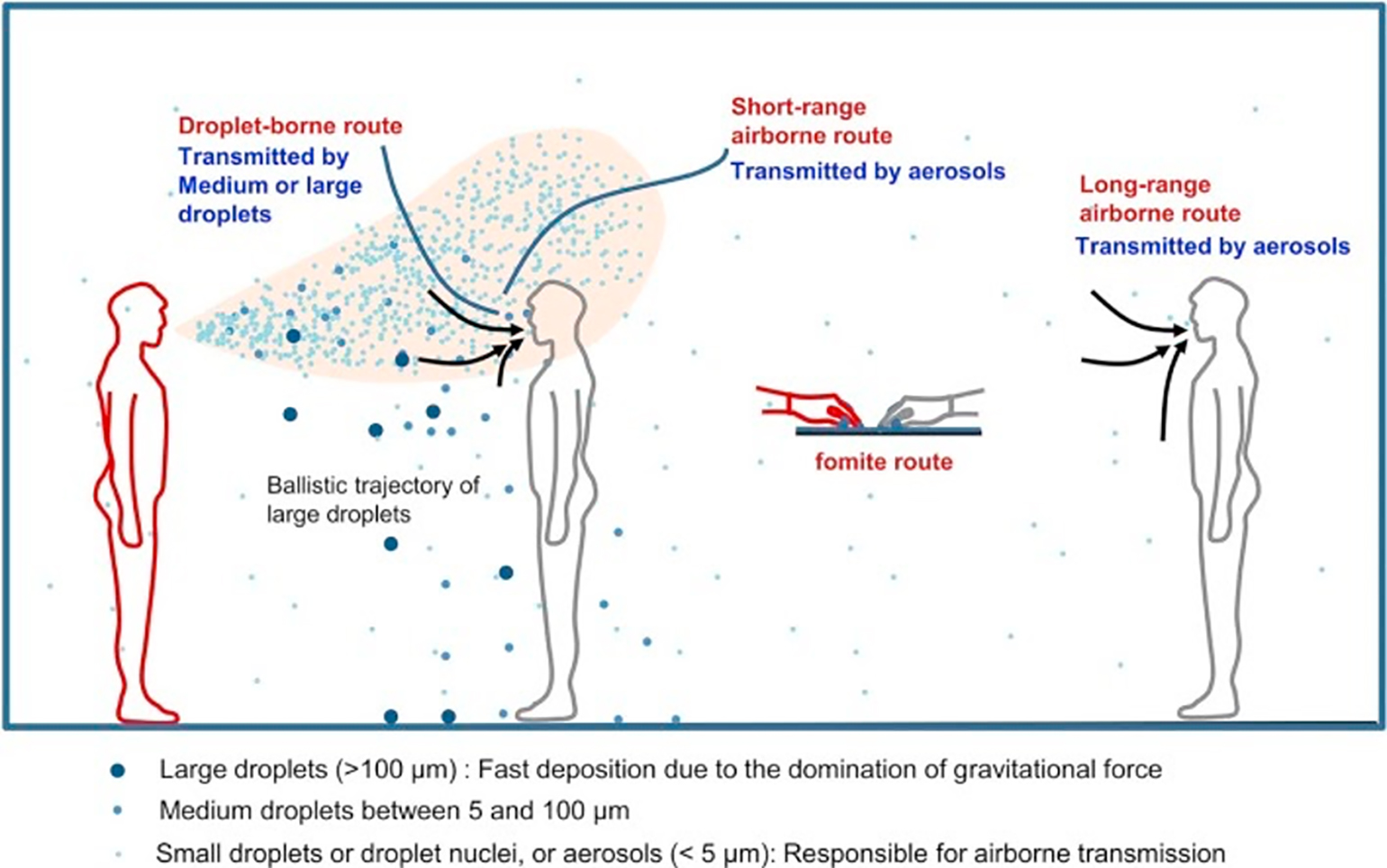
Figure 1
Illustration of different transmission routes (Wei and Li 2016).
A Trip Down Memory Lane: The SARS 2003 Pandemic
SARS transmission occurred predominantly through the droplet and contact routes and did not seem to follow the “classic” long-range airborne route defined by the CDC. However, investigations suggested the possibility of short-range airborne transmission of SARS (Scales et al. 2003; Loeb et al. 2004). Roy and Milton referred to the potential airborne spread of SARS under these specific circumstances as opportunistic — i.e., when a disease that primarily spreads through another mechanism “may use fine-particle aerosols as an efficient means of propagating in favorable environments” (Roy and Milton 2004). An epidemiological analysis of SARS transmission among airplane passengers who shared a flight with an infected person hinted at the plausibility of airborne transmission. However, it was observed that most of the infected persons were seated within three rows (~ 7 feet) of the index patient (Olsen et al. 2003), suggesting this episode of transmission was primarily via large droplets.
The most compelling evidence favoring the possibility of long-range airborne transmission of SARS was developed during a single outbreak at the Amoy Gardens complex in Hong Kong that infected hundreds of residents across neighboring apartment buildings (Yu et al. 2004). Even then, researchers hypothesized that aerosolization of fecal contents may have occurred under this rare circumstance as a result of defective plumbing. For the most part, however, the epidemiological pattern observed during these outbreak investigations -- across small distances and enclosed spaces (Roy and Milton 2004) -- can be explained by transmission through large droplets or fine aerosols, and thus fails to provide definitive evidence of true airborne spread.
Airborne or Not? That is the Question
The “aerobiologic” pathway put forth by Roy and Milton in 2004 (Figure 2) illustrates the the that droplet and aerosol transmission may represent a spectrum of transmission dynamics (Roy and Milton 2004). More importantly, it sheds light on the multitude of factors beyond particle size, such as viral genetics, that affect an organism’s biological and physical decay, and its effective spread via the airborne mechanism (Figure 2). Though recent papers have proposed that the existence of turbulent gas clouds (Bourouiba 2020) and speech particles with an estimated airborne lifetime of 8 to 14 minutes (Stadnytskyi et al. 2020) are worrisome for potential airborne spread of COVID-19, the Roy and Milton framework serves as an important reminder of that presence of airborne particles is not evidence of true airborne transmission of SARS-CoV-2 virus. Establishing airborne transmission requires data to supporting that it remains infective over time or distance.
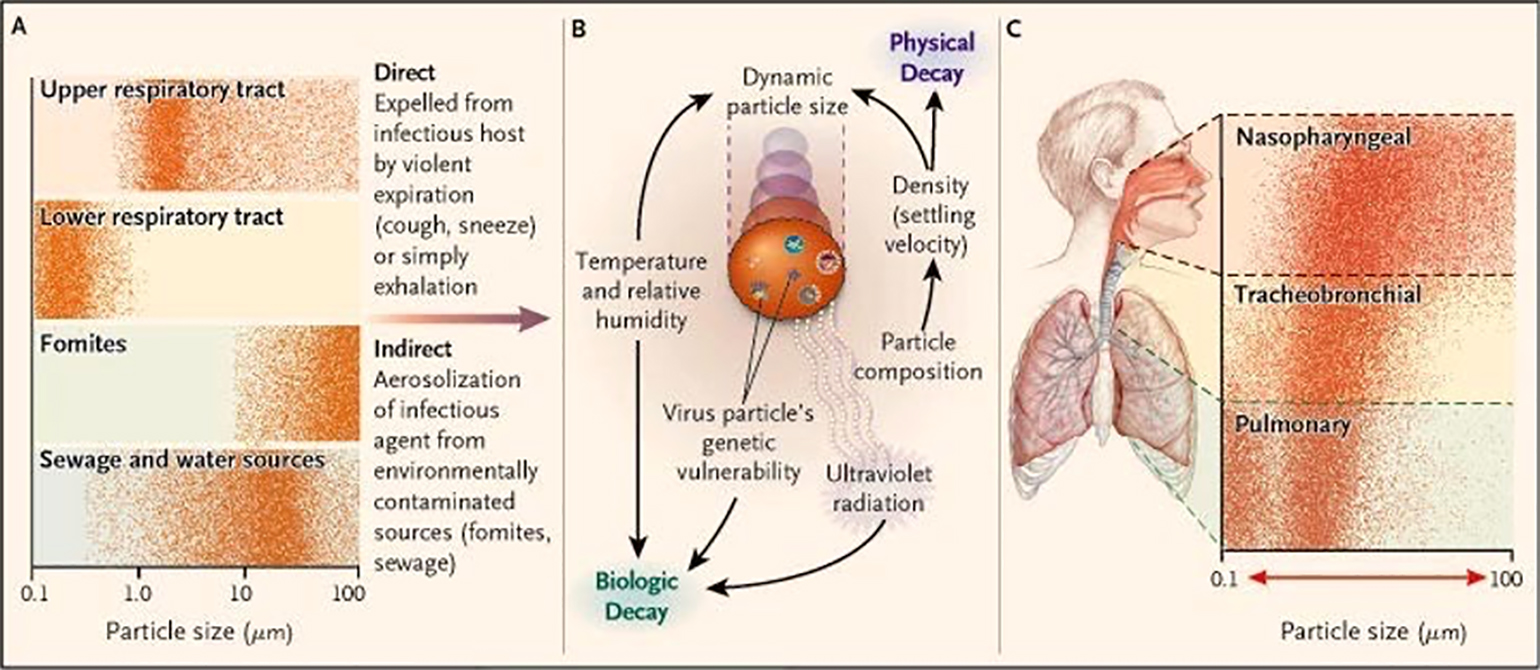
Figure 2
The “aerobiologic pathway” for the transmission of communicable respiratory diseases proposed by Roy and Milton (Roy and Milton 2004).
The rapid spread of COVID-19 across the globe has prompted researchers and public health officials to revisit the age-old question: Is this virus spreading via the airborne route? A letter published in the NEJM reported the three-hour long viability of SARS-CoV-2 in aerosols that were experimentally generated with a nebulizer, though with marked exponential decay in virus titer (van Doremalen et al. 2020). A recent non-peer reviewed preprint from the University of Nebraska found that air sampled in patient rooms and hallways contained SARS-CoV-2 viral RNA; however, further testing did not identify any viable, infectious virus in these samples (Santarpia et al. 2020). Additionally, findings from the Korean CDC have shown that patients who have recovered are not infectious despite some continuing to have positive RT-PCRs (KCDC). These findings reinforce two important notions: 1) that viral RNA is not equivalent to infectious virus, and correspondingly, 2) that the presence of SARS-CoV-2 RNA in air samples does not necessarily imply that this pathogen can be effectively transmitted via the airborne route.
Data thus far analyzing outbreaks and exposures during the care of patients with COVID-19 continue to support droplet and contact routes as the predominant modes of transmission for SARS-CoV-2, mirroring what was observed during the SARS epidemic (CDC: Cluster of Severe Acute Respiratory Syndrome Cases among Protected Health-Care Workers--Toronto, Canada, 2003). For example, an exposure investigation from Hong Kong did not identify any secondary cases among a group of 71 staff and 49 patients in contact with a confirmed COVID-19 patient who was initially admitted under standard precautions and spent thirty-five hours unmasked in an open cubicle (Wong et al. 2020). Investigators credited the lack of nosocomial transmission to the institution’s universal mask policy, aggressive hand hygiene, and environmental cleaning practices (Siegel et al. 2007). A case report from Singapore had similar findings: among 35 healthcare workers wearing surgical masks while in close contact with a COVID-19 positive patient during an aerosol-generating procedure, none tested positive for SARS-CoV-2 two weeks after exposure (Ng et al. 2020). A recently published MMWR report investigated high rates of secondary transmission of COVID-19 among attendees of a choir practice in Washington (Hamner et al. 2020). This superspreading event was heavily featured in media outlets nationwide, raising concerns of airborne spread of COVID-19. Nevertheless, although investigators did raise the possibility of aerosol emission via speech and singing, they concluded that the close and prolonged contact of attendees was highly conducive to disease spread via droplets and fomites.
Conclusions
Although additional studies are needed to shed light on the transmission of SARS-CoV-2, there is strong evidence from the SARS epidemic (Chen et al. 2004; Scales et al. 2003; Seto et al. 2003) and the current COVID-19 pandemic (Wong et al. 2020; Ng et al. 2020; Ran et al. 2020) to suggest that this virus is predominantly transmitted via the droplet and contact routes. There is no evidence that SARS-CoV-2 is an obligate or a preferential aerosol-transmitted airborne disease in the community (Roy and Milton 2004). While nosocomial aerosolization (e.g. with intubation or bronchoscopy) may provide an opportunistic pathway for airborne transmission of SARS-CoV-2, there has been no definitive evidence of airborne transmission of COVID-19. It is important to keep in mind, however, that airborne spread cannot be easily proven via conventional contact tracing and exposure investigations. Novel scientific and epidemiologic approaches that enable the identification of COVID-19 transmission via aerosols or across distance and time may be necessary to reach a more definitive verdict regarding the spread (or lack thereof) of COVID-19 and other respiratory viruses via the airborne route, once and for all.
References
- Bourouiba, Lydia. 2020. “Turbulent Gas Clouds and Respiratory Pathogen Emissions: Potential Implications for Reducing Transmission of COVID-19.” JAMA: The Journal of the American Medical Association, March. https://doi.org/10.1001/jama.2020.4756.
- CDC. 2003. “Cluster of Severe Acute Respiratory Syndrome Cases among Protected Health-Care Workers--Toronto, Canada, April 2003.” MMWR. Morbidity and Mortality Weekly Report 52 (19): 433–36.
- Chen, Yee-Chun, Li-Min Huang, Chang-Chuan Chan, Chan-Ping Su, Shan-Chwen Chang, Ying-Ying Chang, Mei-Ling Chen, et al. 2004. “SARS in Hospital Emergency Room.” Emerging Infectious Diseases 10 (5): 782–88.
- Doremalen, Neeltje van, Trenton Bushmaker, Dylan H. Morris, Myndi G. Holbrook, Amandine Gamble, Brandi N. Williamson, Azaibi Tamin, et al. 2020. “Aerosol and Surface Stability of SARS-CoV-2 as Compared with SARS-CoV-1.” The New England Journal of Medicine 382 (16): 1564–67.
- “From the Centers for Disease Control and Prevention. Cluster of Severe Acute Respiratory Syndrome Cases among Protected Health-Care Workers--Toronto, Canada, April 2003.” 2003. JAMA: The Journal of the American Medical Association 289 (21): 2788–89.
- Hamner, Lea, Polly Dubbel, Ian Capron, Andy Ross, Amber Jordan, Jaxon Lee, Joanne Lynn, et al. 2020. “High SARS-CoV-2 Attack Rate Following Exposure at a Choir Practice - Skagit County, Washington, March 2020.” MMWR. Morbidity and Mortality Weekly Report 69 (19): 606–10.
- KCDC. n.d. “KCDC.” KCDC. Accessed May 31, 2020. http://www.cdc.go.kr.
- Klompas, Michael, Charles A. Morris, Julia Sinclair, Madelyn Pearson, and Erica S. Shenoy. 2020. “Universal Masking in Hospitals in the Covid-19 Era.” The New England Journal of Medicine. https://www.nejm.org/doi/full/10.1056/NEJMp2006372.
- Loeb, Mark, Allison McGeer, Bonnie Henry, Marianna Ofner, David Rose, Tammy Hlywka, Joanne Levie, et al. 2004. “SARS among Critical Care Nurses, Toronto.” Emerging Infectious Diseases 10 (2): 251–55.
- Ng, Kangqi, Beng Hoong Poon, Troy Hai Kiat Puar, Jessica Li Shan Quah, Wann Jia Loh, Yu Jun Wong, Thean Yen Tan, and Jagadesan Raghuram. 2020. “COVID-19 and the Risk to Health Care Workers: A Case Report.” Annals of Internal Medicine, March. https://doi.org/10.7326/L20-0175.
- Olsen, Sonja J., Hsiao-Ling Chang, Terence Yung-Yan Cheung, Antony Fai-Yu Tang, Tamara L. Fisk, Steven Peng-Lim Ooi, Hung-Wei Kuo, et al. 2003. “Transmission of the Severe Acute Respiratory Syndrome on Aircraft.” The New England Journal of Medicine 349 (25): 2416–22.
- Ran, Li, Xuyu Chen, Ying Wang, Wenwen Wu, Ling Zhang, and Xiaodong Tan. 2020. “Risk Factors of Healthcare Workers with Corona Virus Disease 2019: A Retrospective Cohort Study in a Designated Hospital of Wuhan in China.” Clinical Infectious Diseases: An Official Publication of the Infectious Diseases Society of America, March. https://doi.org/10.1093/cid/ciaa287.
- Roy, Chad J., and Donald K. Milton. 2004. “Airborne Transmission of Communicable Infection--the Elusive Pathway.” The New England Journal of Medicine.
- Santarpia, Joshua L., Danielle N. Rivera, Vicki Herrera, M. Jane Morwitzer, Hannah Creager, George W. Santarpia, Kevin K. Crown, et al. 2020. “Transmission Potential of SARS-CoV-2 in Viral Shedding Observed at the University of Nebraska Medical Center.” medRxiv.
- Scales, Damon C., Karen Green, Adrienne K. Chan, Susan M. Poutanen, Donna Foster, Kylie Nowak, Janet M. Raboud, Refik Saskin, Stephen E. Lapinsky, and Thomas E. Stewart. 2003. “Illness in Intensive Care Staff after Brief Exposure to Severe Acute Respiratory Syndrome.” Emerging Infectious Diseases 9 (10): 1205–10.
- Seto, W. H., D. Tsang, R. W. H. Yung, T. Y. Ching, T. K. Ng, M. Ho, L. M. Ho, J. S. M. Peiris, and Advisors of Expert SARS group of Hospital Authority. 2003. “Effectiveness of Precautions against Droplets and Contact in Prevention of Nosocomial Transmission of Severe Acute Respiratory Syndrome (SARS).” The Lancet 361 (9368): 1519–20.
- Shiu, Eunice Y. C., Nancy H. L. Leung, and Benjamin J. Cowling. 2019. “Controversy around Airborne versus Droplet Transmission of Respiratory Viruses: Implication for Infection Prevention.” Current Opinion in Infectious Diseases 32 (4): 372–79.
- Siegel, Jane D., Emily Rhinehart, Marguerite Jackson, Linda Chiarello, Health Care Infection Control Practices Advisory Committee, and Others. 2007. “2007 Guideline for Isolation Precautions: Preventing Transmission of Infectious Agents in Health Care Settings.” American Journal of Infection Control 35 (10): S65.
- Stadnytskyi, Valentyn, Christina E. Bax, Adriaan Bax, and Philip Anfinrud. 2020. “The Airborne Lifetime of Small Speech Droplets and Their Potential Importance in SARS-CoV-2 Transmission.” Proceedings of the National Academy of Sciences of the United States of America, May. https://doi.org/10.1073/pnas.2006874117.
- Tellier, Raymond, Yuguo Li, Benjamin J. Cowling, and Julian W. Tang. 2019. “Recognition of Aerosol Transmission of Infectious Agents: A Commentary.” BMC Infectious Diseases 19 (1): 101.
- Wei, Jianjian, and Yuguo Li. 2016. “Airborne Spread of Infectious Agents in the Indoor Environment.” American Journal of Infection Control 44 (9 Suppl): S102–8.
- Wong, S. C-Y, R. T-S Kwong, T. C. Wu, J. W. M. Chan, M. Y. Chu, S. Y. Lee, H. Y. Wong, and D. C. Lung. 2020. “Risk of Nosocomial Transmission of Coronavirus Disease 2019: An Experience in a General Ward Setting in Hong Kong.” The Journal of Hospital Infection, April. https://doi.org/10.1016/j.jhin.2020.03.036.
- Yu, Ignatius T. S., Yuguo Li, Tze Wai Wong, Wilson Tam, Andy T. Chan, Joseph H. W. Lee, Dennis Y. C. Leung, and Tommy Ho. 2004. “Evidence of Airborne Transmission of the Severe Acute Respiratory Syndrome Virus.” The New England Journal of Medicine 350 (17): 1731–39.
View all COVID-19 Updates
Learn more about research in the Division of Pulmonary and Critical Care Medicine