What Causes Hypoxemia in COVID-19? (Part I)
The FLARE Four
- Critical COVID-19 is characterized by hypoxemic respiratory failure
- The perceived discordance between severity of hypoxemia and both the extent of radiographic disease and level of clinical distress has led many to propose novel and unlikely mechanisms of hypoxemia in COVID-19
- Distinguishing between hypoxemia and true hypoxia is essential to understanding the phenomenon of so-called “happy hypoxics” in COVID-19
- Fundamental mechanisms of hypoxemia are shared by all pulmonary diseases, including COVID-19. The most common causes of severe hypoxemia are low V/Q and its extreme, pulmonary shunt
Many people are saying...hypoxemia in COVID-19 is something entirely new.
Subscribe to the latest updates from FLARE Advances in Motion
Introduction
The canonical presentation of severe COVID-19 is hypoxemia and, often, ARDS (April 10 FLARE). Early studies of gas exchange in ARDS emphasized the predominant contribution of shunt, which is thought to be secondary to continued perfusion of collapsed and/or fluid-filled alveolar units (Albert and Jobe, 2012). The observation of severe hypoxemia in COVID-19 despite only mild parenchymal involvement (Gattinoni et al., 2020) has led some to speculate (Leisman et al., 2020) that novel mechanisms must underlie the hypoxemia. These speculations have been the subject of intense debate both in the scientific literature (Leisman et al., 2020) and in non-peer reviewed forums. Proposed novel mechanisms of hypoxemia include endotheliitis, failure of hypoxic pulmonary vasoconstriction (Archer et al., 2020; Lang et al., 2020) and/or macro- or microvascular thrombosis (Klok et al., 2020).
Tonight, we will review the fundamental mechanisms of hypoxemia, emphasizing V**/Q** mismatch and shunt. Tomorrow, we will address the mechanisms of hypoxemia in ARDS specifically, and discuss whether COVID-19 related hypoxemia (with or without ARDS) requires a novel explanation. To do so, we will evaluate the published observations on hypoxemia in COVID-19. We conclude that, to date, no published observation on COVID-19 related hypoxemia requires the invocation of novel biology specific to SARS-CoV-2.
Hypoxemia and Hypoxia: Are "Happy Hypoxics [sic]" Surprising?
A rigorous exploration of impaired gas exchange in COVID-19 requires precise terminology, and in particular, appropriate use of the terms hypoxia and hypoxemia. Typical measures of gas exchange detect hypoxemia, which is to say a reduced amount of oxygen in the blood. This is appropriately considered to be an alarming clinical finding, but it must be distinguished from the feared consequence of hypoxia – a state of inadequate oxygen supply for tissue needs (NEJM, 1966).
To further clarify the difference between the two terms, consider the differences between oxygen content (CaO2), oxygen delivery (DO2), and oxygen utilization (VO2**) (Gonzalez and Kuwahira, 2011):
- CaO2 = 1.39 x Hgb x SaO2 + 0.003 PaO2
- DO2 = Q x CaO2
- VO2 = Q x (CaO2 - CvO2)
where SaO2 is arterial oxygen saturation, Q is cardiac output, DO2 is actual oxygen delivery, and CvO2 is mixed venous blood oxygen content. From the above, it is apparent that a patient who is hypoxemic (with decreased blood oxygen content from either low arterial saturation or low hemoglobin) can still maintain adequate oxygen delivery (content x cardiac output) by raising cardiac output. Additionally, a patient with reduced oxygen delivery can maintain adequate oxygen consumption by increasing extraction (i.e. by decreasing the mixed venous oxygen content). The fact that oxygen consumption can be maintained (at least at resting levels (Wagner, 1996)) in the setting of hypoxemia - i.e. that hypoxia is not the inevitable result of hypoxemia - likely partially explains the phenomenon of the so-called “happy hypoxic” in COVID-19. As has been reviewed in the April 10th FLARE, hypoxemia itself is a relatively mild stimulus for dyspnea and patients with adequate tissue oxygen supply may not otherwise be in apparent distress despite the steady erosion of their physiologic reserve. Such patients perversely labeled “happy hypoxics” are more properly termed “happy hypoxemics” as they have low SaO2 but do not necessarily have actual tissue hypoxia. Actual tissue hypoxia is neither benign nor a “happy” event.
What Are the Known Causes of Hypoxemia?
The fundamental causes of hypoxemia are common to all cardiopulmonary disease, and each is associated with multiple precipitants. These include: 1) decreased inspired partial pressure of oxygen (PiO2), 2) hypoventilation, 3) V/Q mismatch, 4) shunt, and 5) diffusion limitation (West, 2011). Decreased PiO2 is only relevant to gas exchange at high altitude (West et al., 1962), while diffusion limitation only contributes to hypoxemia under rare circumstances. These include exercise at altitude (Piiper and Scheid, 1981) and exercise in highly trained individuals (Wagner, 1996) or in those with interstitial lung disease (Young and Bye, 2011). Diffusion limitation can also be relevant to gas exchange in an extracorporeal circuit (May 9 FLARE). Due to their limited relevance to commonly seen situations, these two mechanisms will not be discussed further. Additionally, although hypoventilation can contribute to hypoxemia, it is rarely the sole cause of severe hypoxemia and is not a primary mechanism in ARDS. By far, the most common causes of clinical hypoxemia are V/Q mismatch and shunt (in that order).
V/Q Mismatch
Gas exchange across the alveolar-capillary interface occurs by passive diffusion (Kellogg and H., 2011; Wagner, 2015). Therefore, pulmonary capillary blood, and thus arterial blood, cannot have a partial pressure of oxygen (PaO2) that is higher than the alveolar partial pressure of oxygen (PAO2). Assuming that blood in the pulmonary capillary fully equilibrates with the air in the alveolus - i.e. that there is no diffusion limitation - the end-capillary partial pressure of oxygen exactly equals the PAO2. The PaO2 is thus determined by the PAO2. To a first approximation, the PAO2 is determined by amount of blood entering the alveolar unit (the perfusion, Q), the amount of gas entering that alveolar unit (the ventilation, V), and the oxygen present in each (in the blood, oxygen content; in the gas, oxygen partial pressure).
Under most circumstances at rest, neither the inspired partial pressure of oxygen nor the mixed-venous oxygen content is changing significantly and we can therefore assume that the PAO2 is determined entirely by the relative amounts of ventilation and perfusion - the V/Q ratio. If ventilation greatly exceeds perfusion (high V/Q ratio), the PAO2 will be closer to the PiO2. If perfusion greatly exceeds ventilation (low V/Q ratio) the PAO2 will be closer to that of mixed venous blood. In between these two extremes, the PAO2 will adopt intermediate values as depicted in Figure 1. The arterial blood oxygen content is the perfusion-weighted average of the resulting oxygen content from each alveolar unit.
One important point is that high V/Q and low V/Q units will not have an equivalent impact on the arterial content owing to the shape of the hemoglobin saturation curve (Petersson and Glenny, 2014) (Figure 1). Hemoglobin is typically fully saturated at the PAO2 associated with a V/Q of 1 (100 mmHg breathing room air) (Hsia, 1998) -- increases in V/Q above 1 therefore do not significantly increase capillary blood oxygen content, as indicated by the plateau of the green line in the figure. The decreased PAO2 associated with a low V/Q, on the other hand, can lead to big decreases in the capillary oxygen content.
One final point about hypoxemia is an explanation of the so-called “A-a gradient” (the alveolar-arterial oxygen difference (A-a)O2). Many interpret the presence of an (A-a)O2 as evidence of diffusion limitation (i.e. the inability of pulmonary capillary blood to reach diffusive equilibrium with the alveolar space). However, as mentioned above, diffusion limitation is very rare, even in the diseased lung. Instead, in most patients the A-a gradient reflects the presence of low V/Q units within the lung. Each low V/Q unit reaches diffusive equilibrium but because the alveolar space is relatively hypoxic, the resulting capillary blood is relatively hypoxemic. The arterial oxygen content is thus lower than would be predicted if each lung unit had a V/Q ratio of 1 or greater and the patient has an elevated A-a gradient.
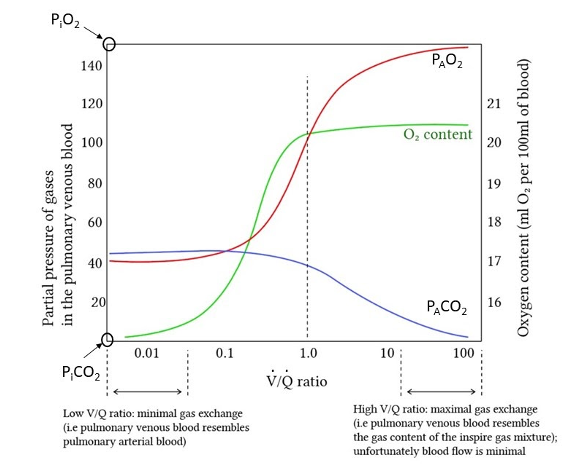
Figure 1
Alveolar partial pressures of CO2 (blue line) and O2 (red line) as a function of V/Q, adapted from Deranged Physiology. As V/Q ratio increases, the alveolar partial pressures approach inhaled partial pressures. As V/Q ratio decreases, the alveolar partial pressures reach mixed venous pressures. Green line indicates associated arterial blood oxygen content.
Shunt
The extreme of low V/Q is shunt. Shunt units are those that receive perfusion but no ventilation -- the pulmonary capillary blood in those units therefore has the same oxygen content as mixed venous blood (Petersson and Glenny, 2014). Hypoxemia due to shunt is unresponsive to increases in PiO2 , since by definition a change in inhaled oxygen content is not experienced by non-ventilated lung units (Scand, 1942). The blood flowing to units with no ventilation simply does not participate in gas exchange, and both enters and exits the lungs with the same oxygen content as mixed venous blood.
The Effect of Low V/Q and Shunt on Arterial Oxygenation
Understanding this basic physiology is highly clinically relevant since patients with opacities on chest imaging, such as those with COVID-19, can be presumed to have areas of impaired ventilation. Importantly, the impact of impaired ventilation on arterial oxygenation will depend on the perfusion to those areas and therefore the extent of imaging abnormality will not reliably correlate with the degree of hypoxemia.
Blood flowing from low V/Q units will be fully equilibrated with the alveolar oxygen in those units (via diffusive equilibrium), but due to the lower PAO2 will have a lower oxygen content than blood flowing from normal or high V/Q units. Blood coming from shunt units will have the oxygen content of venous blood. Mixed arterial oxygen content will be a perfusion-weighted average of oxygen content of both the blood volume that has perfused shunt units and the volume of blood that has perfused lung units with V/Q ratios spanning the entire spectrum from low to high. Shunt and low V/Q thus lower mixed arterial (i.e. systemic) blood oxygen content, resulting in hypoxemia (Figure 2).
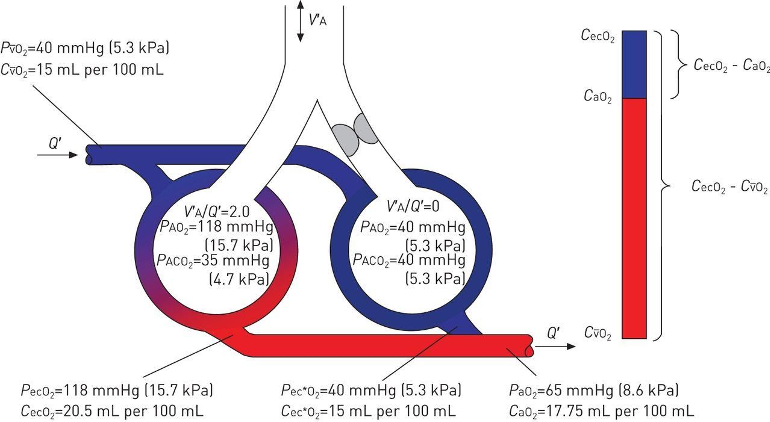
Figure 2
The effect of low V/Q and shunt units on arterial oxygen content. (Petersson and Glenny, 2014)
Conclusion
The most common cause of significant hypoxemia is low V/Q and its lower extreme, shunt. Lung units with a V/Q ratio < 1 have concomitantly lower alveolar partial pressure of oxygen, and therefore the pulmonary capillary blood from those units is also relatively hypoxemic. Heterogeneity of V/Q, i.e. the distribution of lung units across the V/Q spectrum, is a hallmark of lung disease, and arterial oxygen content is determined by this heterogeneity and the amount of perfusion to each unit. Tomorrow’s FLARE will address mechanisms of hypoxemia in ARDS and the ability of hypoxic pulmonary vasoconstriction to compensate for pulmonary disease. We will also examine whether COVID-19 related hypoxemia requires novel explanations. Through this approach, we will refute the notion that hypoxemia in COVID-19 is caused by a new, unexplained entity.
** notable abbreviations: V = V dot, Q = Q dot, VO2 = V(dot)O2.
References:
- Albert, R.K., and Jobe, A. (2012). Gas Exchange in the Respiratory Distress Syndromes. In Comprehensive Physiology, R. Terjung, ed. (Hoboken, NJ, USA: John Wiley & Sons, Inc.), p. 818.
- Archer, S.L., Sharp, W.W., and Weir, E.K. (2020). Differentiating COVID-19 Pneumonia from Acute Respiratory Distress Syndrome (ARDS) and High Altitude Pulmonary Edema (HAPE): Therapeutic Implications. Circulation.
- Gattinoni, L., Chiumello, D., Caironi, P., Busana, M., Romitti, F., Brazzi, L., and Camporota, L. (2020). COVID-19 pneumonia: different respiratory treatments for different phenotypes? Intensive Care Med.
- Gonzalez, N.C., and Kuwahira, I. (2011). Systemic Oxygen Transport with Rest, Exercise, and Hypoxia: A Comparison of Humans, Rats, and Mice. In Comprehensive Physiology, D.M. Pollock, ed. (Hoboken, NJ, USA: John Wiley & Sons, Inc.), pp. 1537–1573.
- Hsia, C.C. (1998). Respiratory function of hemoglobin. N. Engl. J. Med. 338, 239–247.
- Kellogg, and H., R. (2011). Laws of Physics Pertaining to Gas Exchange. In Comprehensive Physiology, R. Terjung, ed. (Hoboken, NJ, USA: John Wiley & Sons, Inc.), p. 249.
- Klok, F.A., Kruip, M.J.H.A., van der Meer, N.J.M., Arbous, M.S., Gommers, D.A.M.P.J., Kant, K.M., Kaptein, F.H.J., van Paassen, J., Stals, M.A.M., Huisman, M.V., et al. (2020). Incidence of thrombotic complications in critically ill ICU patients with COVID-19. Thromb. Res.
- Lang, M., Som, A., Mendoza, D.P., Flores, E.J., Reid, N., Carey, D., Li, M.D., Witkin, A., Rodriguez-Lopez, J.M., Shepard, J.-A.O., et al. (2020). Hypoxaemia related to COVID-19: vascular and perfusion abnormalities on dual-energy CT. Lancet Infect. Dis.
- Leisman, D.E., Deutschman, C.S., and Legrand, M. (2020). Facing COVID-19 in the ICU: vascular dysfunction, thrombosis, and dysregulated inflammation. Intensive Care Med.
- NEJM (1966). Hypoxemia vs. hypoxia. N. Engl. J. Med. 274, 908–909.
- Petersson, J., and Glenny, R.W. (2014). Gas exchange and ventilation-perfusion relationships in the lung. Eur. Respir. J. 44, 1023–1041.
- Piiper, J., and Scheid, P. (1981). Model for capillary-alveolar equilibration with special reference to O2 uptake in hypoxia. Respir. Physiol. 46, 193–208.
- Scand, A.P. (1942). The Oxygen Content of Arterial Blood when breathing Air and inhaling Oxygen. Acta Physiol. Scand. 4, 80–83.
- Wagner, P.D. (1996). Determinants of maximal oxygen transport and utilization. Annu. Rev. Physiol. 58, 21–50.
- Wagner, P.D. (2015). The physiological basis of pulmonary gas exchange: implications for clinical interpretation of arterial blood gases. Eur. Respir. J. 45, 227–243.
- West, J.B. (2011). Causes of and Compensations for Hypoxemia and Hypercapnia. In Comprehensive Physiology, R. Terjung, ed. (Hoboken, NJ, USA: John Wiley & Sons, Inc.), p. 886.
- West, J.B., Lahiri, S., Gill, M.B., Milledge, J.S., Pugh, L.G., and Ward, M.P. (1962). Arterial oxygen saturation during exercise at high altitude. J. Appl. Physiol. 17, 617–621.
- Young, I.H., and Bye, P.T.P. (2011). Gas Exchange in Disease: Asthma, Chronic Obstructive Pulmonary Disease, Cystic Fibrosis, and Interstitial Lung Disease. In Comprehensive Physiology, R. Terjung, ed. (Hoboken, NJ, USA: John Wiley & Sons, Inc.), pp. 663–697.
Learn more about research in the Division of Pulmonary and Critical Care Medicine
View all COVID-19 Updates