Is COVID-19 ARDS?
The FLARE Four
- It has been alleged that COVID-19 associated respiratory failure is the result of a novel pathophysiology and is therefore not ARDS
- Such sentiments are frequently based on a misunderstanding of the definition of ARDS, its spectrum of disease, optimal treatment and the physiology of hypoxemia
- Almost all commentators, even those who argue it is not ARDS, appear to agree that large distending pressures in the inflamed lung can be injurious and lead to worsening of gas exchange and pulmonary mechanics. This is the essential pathophysiology of ARDS
- COVID-19 associated respiratory failure is characterized clinically by hypoxemia and bilateral infiltrates, pathologically by diffuse alveolar damage and is optimally treated by a strategy that minimizes distending alveolar pressure and lung stretch. On this basis, COVID-19 associated respiratory failure is ARDS
Subscribe to the latest updates from FLARE Advances in Motion
This piece is courtesy of Dr. Corey Hardin.
Many people are saying...COVID-19 associated respiratory failure is not ARDS.
There is Concern that COVID-19 Associated Respiratory Failure is Not ARDS Because….
The arguments in favor of COVID-19 associated respiratory failure being distinct from ARDS have largely been made in informal arenas such as on social media and in the popular press. As such, they are frequently lacking in the precision and detail required for analysis and appraisal. Exceptions are a recent letter to the American Journal of Respiratory and Critical Medicine (Gattinoni et al., 2020a) and an editorial in Intensive Care Medicine (Gattinoni et al., 2020b). These arguments have generated a great deal of attention, but are not supported by data or in-depth explanation. As best as we can determine, the argument is based on the observations that in some cases:
- Imaging abnormalities in COVID-19 are mild
- Pulmonary mechanical abnormalities (respiratory system compliance, Crs) are mild
- Hypoxemia is out of proportion to either the mechanical abnormalities or to the level of dyspnea
In evaluating these observations, we must ask two questions:
- First, given that most reports of the above are anecdotal, do they accurately reflect the published experience of COVID-19 associated respiratory failure?
- Second, if true, would they constitute an atypical presentation of ARDS?
To the first question, we have recently reviewed (April 8 FLARE) the published case series from China, Italy, and Seattle on critically ill patients with ARDS (Bhatraju et al., 2020; Grasselli et al., 2020; Yang et al., 2020). These data reveal a wide spread in the disease severity of COVID-19 with a corresponding spectrum of gas exchange impairments and changes in respiratory system compliance. An initial report of 138 patients from China (Wang et al., 2020) found a median P:F of 136 (which would be consistent with moderate ARDS), but with a range that extended from severe to mild (103-234). Reports from Washington state were also notable for a variety of severities with a median P:F of 142 (range 94-177) and a median decreased respiratory system compliance of 29 mL/cm H2O (25-36) on day 1. Finally, the large Italian series reviewed by FLARE this week (April 8 FLARE) had a mean P:F of 160 (range 114-220).
These data compare to a median respiratory system compliance of 30 mL/cm H2O in PROSEVA, a multi-center ARDS study in the pre-COVID-19 era (Guérin et al., 2013) and a mean P:F of 161 in LUNG-SAFE, a contemporary snapshot of ARDS of multiple etiologies (Bellani et al., 2016). In sum, there do appear to be a fairly significant number of COVID-19 patients presenting with mild derangements of respiratory system compliance and gas exchange. However, the degree of these abnormalities is well within the spectrum of disease expected based on experience with ARDS prior to advent of COVID-19.
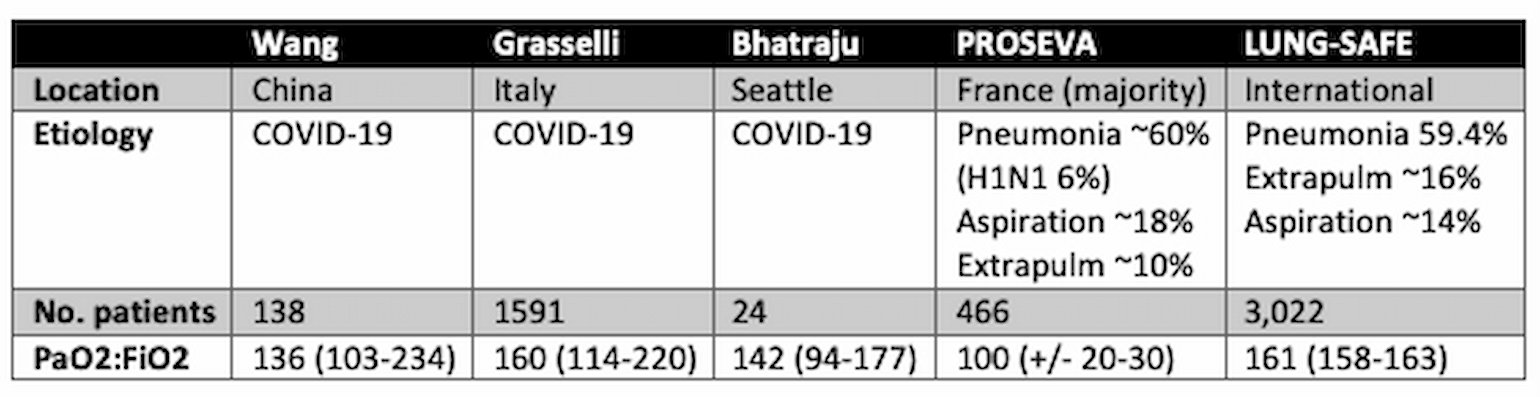
Table 1
NR = not reported. N.b. PROSEVA was a trial which included only patients with P:F <150, therefore these data are enriched for patients with severe ARDS.
The third observation cited above is more difficult to analyze, because there are, at the time of this writing, no published reports of the phenomenon and no effort in the anecdotes to quantify what is meant by “hypoxemia out of proportion to dyspnea”. To the extent that this is a real phenomenon, it is important to note that dyspnea has a fairly complex relationship to hypoxemia in most lung disease (Manning and Schwartzstein, 1995). Dr. Richard Schwartzstein, Chief of the Division of Pulmonary and Critical Care at Beth Israel Deaconess Medical Center in Boston, MA, and author of the cited manuscript, when asked about this observation, points out the following (personal communication):
- Hypoxemia alone is a relatively weak stimulus for dyspnea and ventilation at rest. Physiologically/teleologically that makes sense in a way because increasing ventilation does relatively little to improve O2 content, given the shape of the O2/Hgb saturation curve, until PaO2 is below 60 and even then, it is a modest stimulus (VE at PO2 45 is about 10L/min in healthy people).
- There is inter-individual variation in the “sensitivity” of the chemoreceptors and breathing discomfort among normal individuals and in disease states (think “blue bloaters” vs “pink puffers”).
- Dyspnea in most patients with lung disease arises because of stimulation of pulmonary afferents that either directly produce a sensation (e.g., chest tightness in asthma), stimulate the respiratory controller and lead to a sensation of “air hunger,” or put a load on the ventilatory pump (increased “work” of breathing), or weakened ventilatory muscles (increased “effort” to breathe). Air hunger is made much more intense if tidal volume is restricted by disease physiology (think dynamic hyperinflation in COPD with reduced inspiratory capacity) or by iatrogenic means (e.g., low tidal volume ventilation).
Given the relatively mild mechanical deficits described above, a phenotype with relatively mild dyspnea should be expected. It is also possible that there are subsets of COVID-19 which are indeed characterized by low V/Q due to perfusion deficits without much parenchymal injury. As Dr. Schwartzstein further comments: “if a patient had primarily a vascular endothelial injury leading to V/Q mismatch, that may be less dyspnogenic.” In that context, it is important to note that endothelial injury and pulmonary vascular disruption have long been observed in ARDS (Naeije and Brimioulle, 2001). Greene and colleagues, nearly 35 years ago, performed pulmonary angiography (Greene, 1986) in patients with ARDS and found significant incidence of endothelial injury, vascular obstruction and clot, the degree of which was correlated with outcome.
In answer to the questions posed above, then, some of the anecdotal observations are consistent with published data (mild gas exchange abnormalities, modest impairments in mechanics)—that’s true—but they are also consistent with observations in large ARDS studies in the pre-COVID-19 era.
What is ARDS? Do patients with COVID-19-associated Acute Respiratory Failure Fit Accepted Diagnostic Criteria?
There are a number of ways to answer this question - clinically, pathologically and with reference to pathophysiology.
Clinical
ARDS may be diagnosed using well-known clinical definitions such as the Berlin criteria (ARDS Definition Task Force et al., 2012) promoted by the NIH funded Prevention and Early Treatment of Acute Lung Injury Network (PETAL). We have already reviewed the data on mean P:F in the COVID-19 case series to date. Here we will add that the vast majority of patients in published case series are intubated (88% in Italian series) (Grasselli et al., 2020) and thus on PEEP, and virtually all patients in published series were characterized by bilateral infiltrates (100% in Seattle series, 80-100% in Chinese series (Guan et al., 2020; Wang et al., 2020). On this basis, it can be said that patients with hypoxemia in association with COVID-19 almost universally meet diagnostic criteria for ARDS.
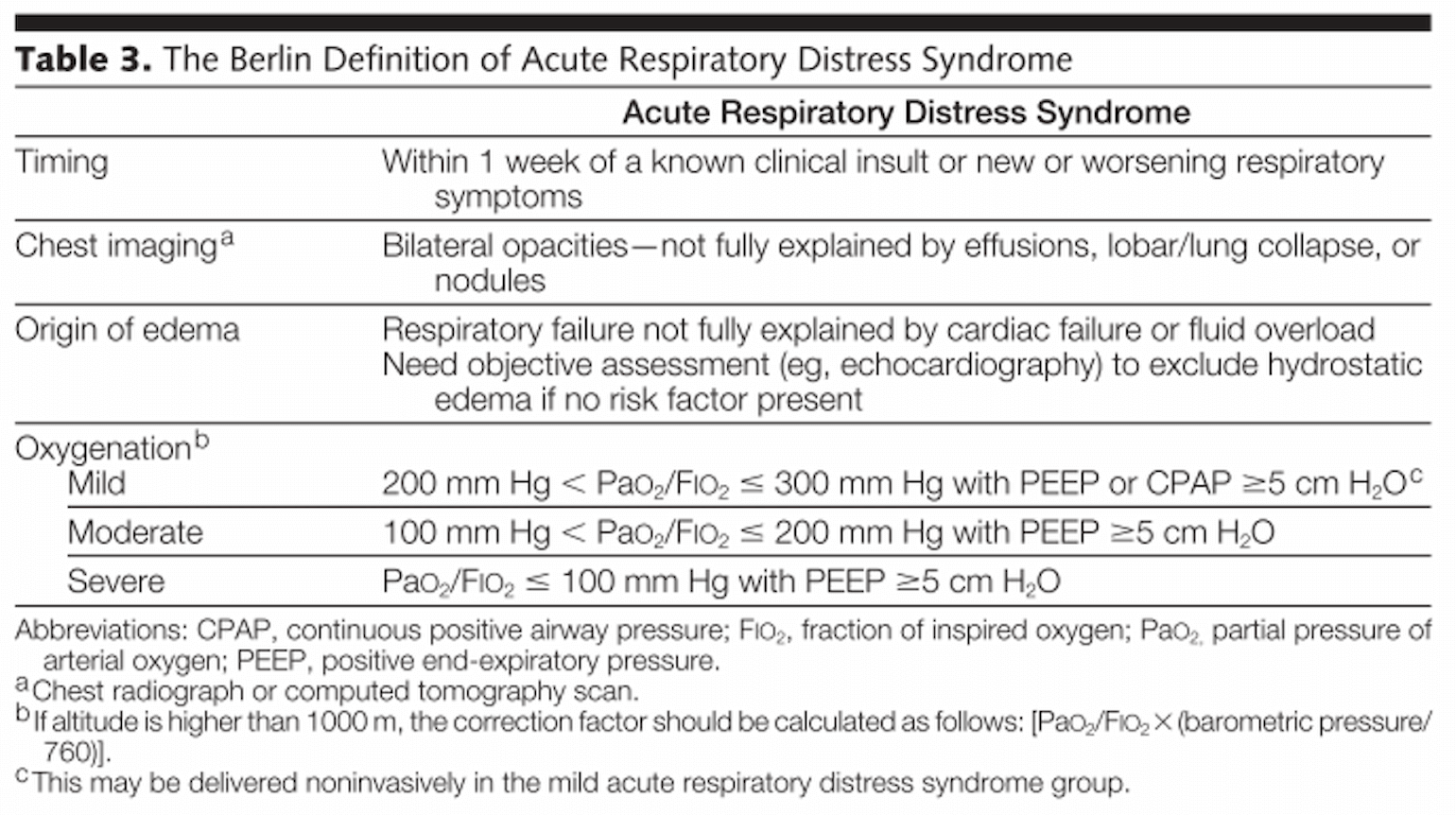
Figure 1
(ARDS Definition Task Force et al., 2012).
Limitations to Berlin Criteria
The Berlin criteria for ARDS have been criticized as being overly inclusive (Villar et al., 2016) and lacking specificity as to the degree of lung injury. Nevertheless, the range of mechanical and gas exchange abnormalities exhibited by patients with COVID-19 were, as detailed above, within the range of those exhibited by patients enrolled in prior ARDS trials. To the extent that patients with COVID-19 associated respiratory failure meet the Berlin criteria for ARDS, they are similar to patients enrolled in ARDS trials based on the same criteria. Therefore, we should be confident in applying the results of those trials to our current COVID-19 patients.
Pathology
Aside from clinical definitions, ARDS is characterized pathologically by diffuse alveolar damage (DAD), a pathologic pattern notable for hyaline membrane formation, lung edema, inflammation, hemorrhage, and alveolar epithelial cell injury (Tomashefski, 2000). This early in the outbreak pathology samples are limited. However, available data demonstrate a predominance of DAD (Xu et al., 2020; Zhang et al., 2020).
The Pathophysiology of ARDS is also the Pathophysiology of Much Hypoxemic Respiratory Failure
Physiologically, there are five causes of hypoxemia and only two common ones in hypoxemic respiratory failure -- shunt and V/Q mismatch (Hardin et al., 2019). For this reason, there is some overlap in the pathophysiology of ARDS and other diseases which result in hypoxemia. Hypoxemia in ARDS is due to shunt and V/Q mismatch. A primary mechanism (Fig. 1) leading to shunt and V/Q mismatch in ARDS is alveolar instability. Alveolar instability results from surfactant inactivation, which occurs in the setting of increased permeability of the alveolar capillary membrane and extravasation of fluid and proteins into the airspaces. Without surfactant, alveoli need greater transpulmonary pressures to remain inflated, and without these pressures they remain underinflated or collapse—contributing to areas of V/Q mismatch or shunt. The application of PEEP can promote reinflation of collapsed alveoli (called recruitment).
Crucially, the disease is heterogeneous; while recruitment maneuvers may improve shunt or V/Q mismatch in some lung units, they may also cause over-distention injury in already well-inflated lung units (barotrauma). For this reason, ventilatory strategies in ARDS stress low tidal volumes (since full volume is potentially going only to the well inflated lung) and cautious use of PEEP (see April 2nd FLARE) (Thompson et al., 2017). Lung protective ventilation is gentle ventilation.
In addition to the risk of over-distending good lung, lung injury may be caused purely by non-uniform inflation. At the junction between well-inflated and collapsed lung, the transpulmonary pressure is multiplied (Mead et al., 1970). Thus in the setting of heterogeneous inflation, even normal transpulmonary pressure will be increased at the site of stress-multipliers, and may become injurious.
There is nothing entirely specific to ARDS in the above sequence of events. Localized inflammation, leading to heterogeneous alveolar instability may be present in ARDS, community acquired pneumonia, aspiration, trauma, and, perhaps to a lesser extent High Altitude Pulmonary Edema. This is plausibly the reason why low tidal ventilation benefits virtually all patients on mechanical ventilators – regardless of reason for ventilation (Davies et al., 2016; Futier et al., 2013). Indeed, non-ARDS hypoxemic respiratory failure may evolve into hypoxemic respiratory failure by the use of large tidal volumes (Gajic et al., 2004).
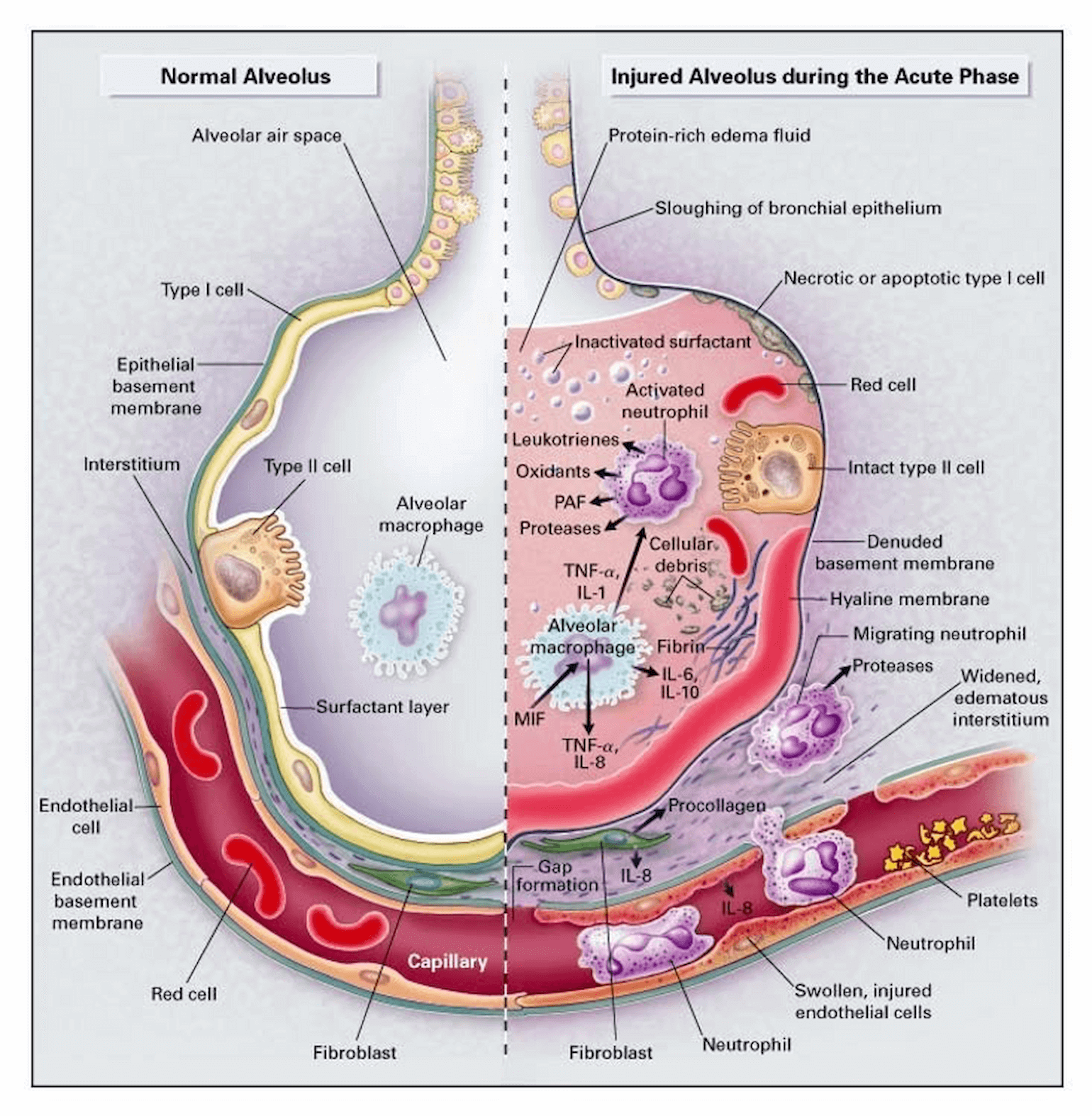
Figure 2
Mechanism of alveolar instability in ARDS: Increased permeability of alveolar-capillary interface leads to surfactant dysfunction and subsequent increased opening pressure and decreased Crs (Ware and Matthay, 2000).
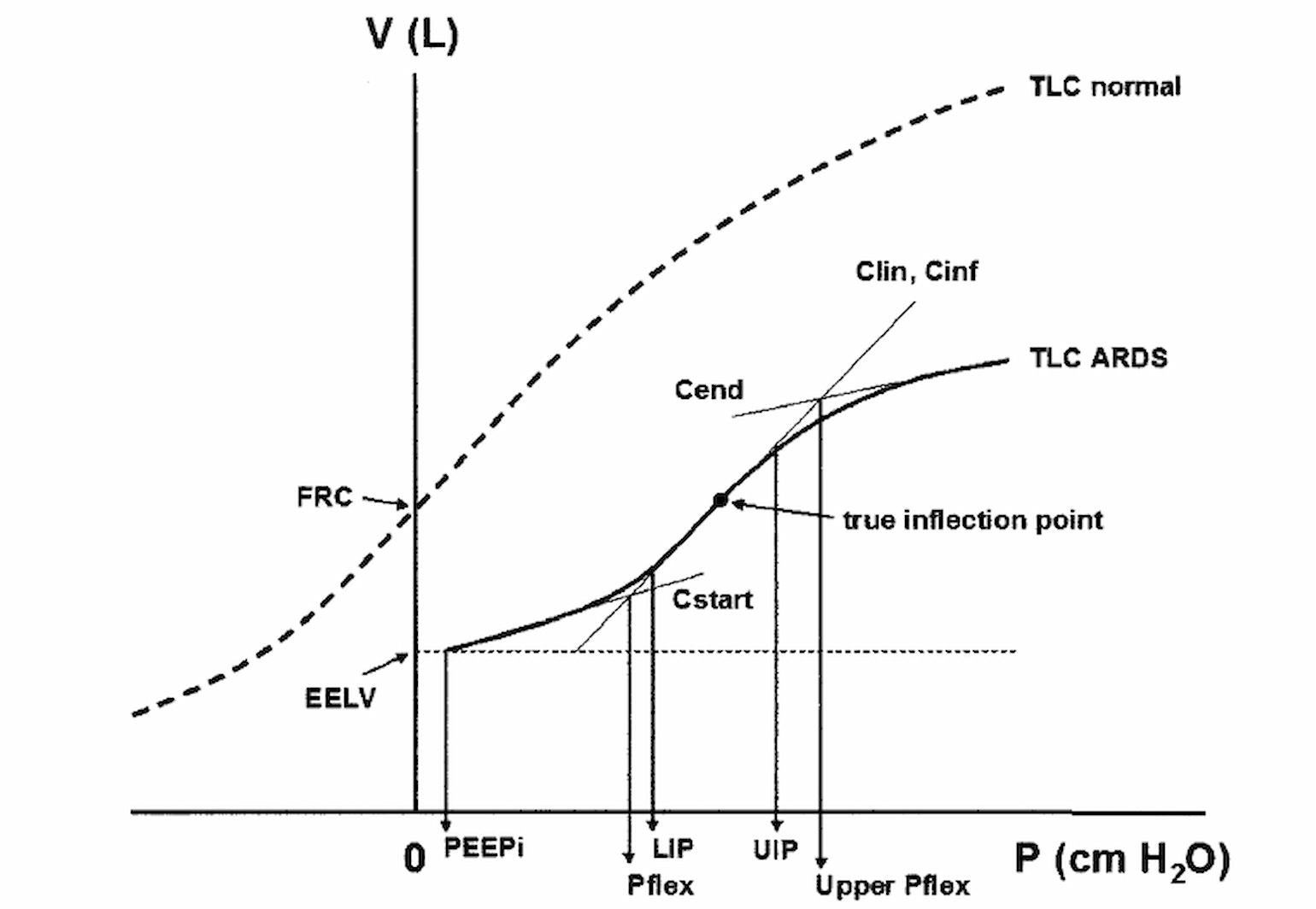
Figure 3
Mechanism of alveolar instability in ARDS: The disease is typically heterogeneous so that attempts to recruit some units (low on PV curve) overdistended well inflated units (high on PV curve) and thus further propagates lung injury (Harris, 2005).
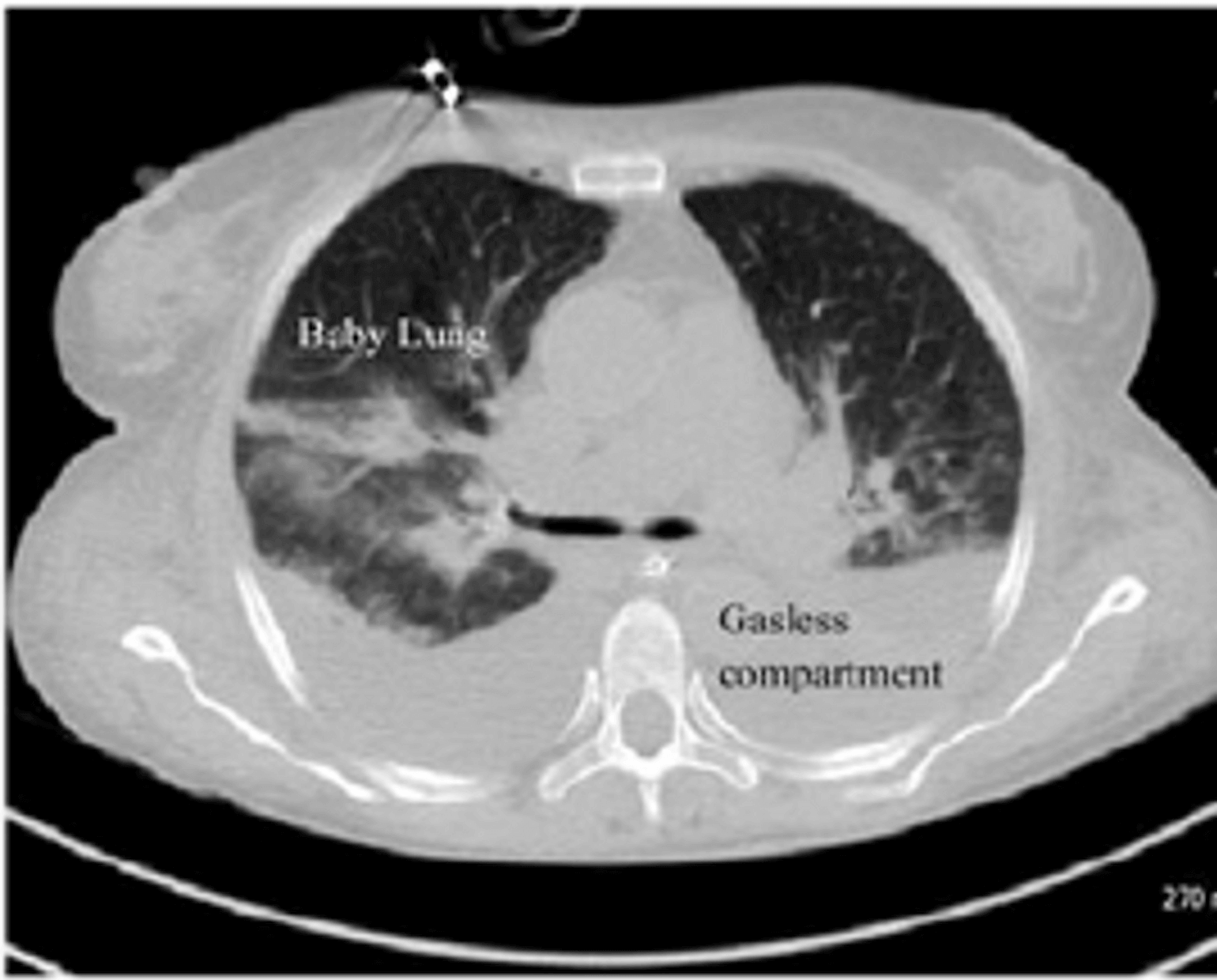
Figure 4
Mechanism of alveolar instability in ARDS: In the setting of complete collapse of some lung regions, the remaining, smaller lung (the so-called ‘baby lung’) may become overdistended by normal tidal volumes (Gattinoni et al., 2016).
How Do People Propose that COVID-19 Pathophysiology Differs from ARDS?
To support the contention that COVID-19 associated respiratory failure is distinct from ARDS, various alternative mechanisms have been proposed. These are not always as distinct from the above sequence of events as their proponents maintain.
High Altitude Pulmonary Edema
In response to the observation (not yet documented in the published literature) that patients with COVID-19 have hypoxemia out of proportion to dyspnea, some propose that hypoxemia is caused by pathologic alterations in the distribution of pulmonary perfusion, rather than alveolar instability. For that reason, some allege that COVID 19-is analogous to High Altitude Pulmonary Edema (HAPE). HAPE is thought to be a disorder of hypoxemic vasoconstriction (Swenson and Bärtsch, 2012). In normal circumstances, alveolar hypoxemia results in local vasoconstriction so that blood is redirected to less hypoxemic, better ventilated parts of the lung thus reducing V/Q mismatch. People susceptible to HAPE are thought to have a hypoxic ventilatory response (HVR) that varies throughout the lung so that in the setting of widespread hypoxemia (i.e. at altitude) some areas of the lung with a relatively impaired HVR receive an overwhelming portion of the cardiac output causing rupture of the capillaries and alveolar edema. In COVID-19, it is proposed that a similar maldistribution of pulmonary perfusion results in areas of low ventilation (due to edema) and high perfusion (due to the redirection of cardiac output). Aside from being entirely unproven, such a mechanism would still result in local edema (perhaps explaining the radiographic abnormalities), heterogeneous alveolar collapse, and stress multipliers. It would, in fact recreate the same pathologic cascade as described in ARDS above and would be expected to respond to the same treatment.
Hemoglobin Binding Dysregulation
A proposed mechanism that has received a lot of attention on social media but is definitively not playing a role in COVID-19 is a direct viral-mediated attack on the hemoglobin molecule. This hypothesis was advanced in an article posted to a chemistry pre-print server (ChemRxiv) and commented on by twitter users and journalists (see here and here). The original paper by Liu and colleagues is a biophysical modeling study which finds sequence homology between SARS-CoV-2 structural proteins and known hemoglobin binding proteins. The authors go on to construct a computer model of binding of these proteins to hemoglobin and suggest that such binding could interfere with oxygen transport. While hypothesis generating, such a mechanism would completely fail to explain the decreased P:F in COVID-19. The P:F is the ratio of PaO2, the partial pressure of arterial blood, to the fraction of inspired oxygen. The partial pressure of arterial blood is the amount of oxygen directly dissolved in the blood - i.e. not bound to hemoglobin. No amount of interference with the hemoglobin molecule will affect the amount of dissolved oxygen.
Distinct Phenotypes: Late Intubation, Non-Invasive Support and Large Tidal Volumes
Without speculating on precise mechanism, Gatinonni et al. suggested that COVID-19 presents with distinct phenotypes at least one of which (Type ‘L’) is entirely distinct from ARDS while a second (type ‘H’) is essentially severe ARDS with widespread alveolar collapse. Since it is believed to not be ARDS, they propose that type ‘L’ could therefore be treated with large tidal volumes (irrespective of the literature cited above which demonstrates a benefit of low tidal volume ventilation in non-ARDS hypoxemic respiratory failure).
Gattinoni and colleagues argue that the ability to tolerate large tidal volumes (which they associate with type ‘L’) means that such patients would benefit from noninvasive respiratory support (Gattinoni et al., 2020b). Non-invasive respiratory support is frequently avoided in ARDS due the risk of causing further lung injury via increased tidal volumes. Increased tidal volumes, in this setting, occur when the dyspneic patient, with high respiratory drive, vigorously contracts the diaphragm, generating large negative pleural pressures and therefore large trans-pulmonary pressures (transpulmonary pressure = pressure at the airway opening - pleural pressure). For this reason, early intubation has been advocated in order to limit distending pressures. In fact, this strategy has been widely employed in the COVID-19 outbreak. Gattinoni and colleagues counter that since the lungs in many COVID-19 patients are not that stiff, they can tolerate large transpulmonary pressures and subsequent large tidal volumes. We worry that this hypothesis ignores decades of evidence for lung-protective ventilation, including in non-ARDS hypoxemic respiratory failure. Gattinoni and colleagues in fact, acknowledge that large transpulmonary pressures can cause progression from type L to severe ARDS (Type ‘H’):
“the possible key feature which determines the evolution of the disease - other than the severity of the disease itself, is the depth of the negative intrathoracic pressure associated with the increased tidal volume in spontaneous breathing. Indeed, the combination of a negative inspiratory intrathoracic pressure and increased lung permeability due to inflammation, results in interstitial lung edema... recently recognized as the leading cause of Patient - Self Inflicted Lung Injury (P-SILI).”
(Gattinoni et al., 2020b)
The authors are therefore in the perverse position of insisting that the pathophysiology of ARDS is present, but arguing for the abandonment of the most successful evidence-based treatment for ARDS (Acute Respiratory Distress Syndrome Network et al., 2000). We are unable to endorse this logic.
Conclusion
ARDS is a heterogeneous disease. It presents with a wide range of severity, varying degrees of hypoxemia and varying degrees of pulmonary mechanical impairment. Failure of hypoxemic vasoconstriction (Naeije and Brimioulle, 2001), alveolar collapse, low V/Q, vascular obstruction and shunt are likely present to varying degrees in any unselected cohort of patients. Nevertheless, there is a common pathway of inflammation, then localized alveolar collapse leading to simultaneous over-distension (of the remaining “baby lung”) and need for recruitment (of the collapsed lung). Diseases in which this pathologic sequence plays out are characterized clinically by hypoxemia and bilateral infiltrates, pathologically by diffuse alveolar damage and are optimally treated by a strategy that minimizes distending pressure and lung stretch. COVID-19 is such a disease and on that basis, it is ARDS.
References
- Acute Respiratory Distress Syndrome Network, Brower, R.G., Matthay, M.A., Morris, A., Schoenfeld, D., Thompson, B.T., and Wheeler, A. (2000). Ventilation with lower tidal volumes as compared with traditional tidal volumes for acute lung injury and the acute respiratory distress syndrome. N. Engl. J. Med. 342, 1301–1308.
- ARDS Definition Task Force, Ranieri, V.M., Rubenfeld, G.D., Thompson, B.T., Ferguson, N.D., Caldwell, E., Fan, E., Camporota, L., and Slutsky, A.S. (2012). Acute respiratory distress syndrome: the Berlin Definition. JAMA 307, 2526–2533.
- Bellani, G., Laffey, J.G., Pham, T., Fan, E., Brochard, L., Esteban, A., Gattinoni, L., van Haren, F., Larsson, A., McAuley, D.F., et al. (2016). Epidemiology, Patterns of Care, and Mortality for Patients With Acute Respiratory Distress Syndrome in Intensive Care Units in 50 Countries. JAMA 315, 788–800.
- Bhatraju, P.K., Ghassemieh, B.J., Nichols, M., Kim, R., Jerome, K.R., Nalla, A.K., Greninger, A.L., Pipavath, S., Wurfel, M.M., Evans, L., et al. (2020). Covid-19 in Critically Ill Patients in the Seattle Region - Case Series. N. Engl. J. Med.
- Davies, J.D., Senussi, M.H., and Mireles-Cabodevila, E. (2016). Should A Tidal Volume of 6 mL/kg Be Used in All Patients? Respir. Care 61, 774–790.
- Futier, E., Constantin, J.-M., Paugam-Burtz, C., Pascal, J., Eurin, M., Neuschwander, A., Marret, E., Beaussier, M., Gutton, C., Lefrant, J.-Y., et al. (2013). A trial of intraoperative low-tidal-volume ventilation in abdominal surgery. N. Engl. J. Med. 369, 428–437.
- Gajic, O., Dara, S.I., Mendez, J.L., Adesanya, A.O., Festic, E., Caples, S.M., Rana, R., St. Sauver, J.L., Lymp, J.F., Afessa, B., et al. (2004). Ventilator-associated lung injury in patients without acute lung injury at the onset of mechanical ventilation*. Read Online: Critical Care Medicine | Society of Critical Care Medicine 32, 1817.
- Gattinoni, L., Marini, J.J., Pesenti, A., Quintel, M., Mancebo, J., and Brochard, L. (2016). The “baby lung” became an adult. Intensive Care Med. 42, 663–673.
- Gattinoni, L., Coppola, S., Cressoni, M., Busana, M., and Chiumello, D. (2020a). Covid-19 Does Not Lead to a “Typical” Acute Respiratory Distress Syndrome. Am. J. Respir. Crit. Care Med.
- Gattinoni, L., Chiumello, D., Caironi, P., Busana, M., Romitti, F., Brazzi, L., Camporota, L., and Gattinoni, L. (2020b). COVID-19 pneumonia: different respiratory treatment for different phenotypes?
- Grasselli, G., Zangrillo, A., Zanella, A., Antonelli, M., Cabrini, L., Castelli, A., Cereda, D., Coluccello, A., Foti, G., Fumagalli, R., et al. (2020). Baseline Characteristics and Outcomes of 1591 Patients Infected With SARS-CoV-2 Admitted to ICUs of the Lombardy Region, Italy. JAMA.
- Greene, R. (1986). Pulmonary vascular obstruction in the adult respiratory distress syndrome. Journal of Thoracic Imaging 1, 31–38.
- Guan, W.-J., Ni, Z.-Y., Hu, Y., Liang, W.-H., Ou, C.-Q., He, J.-X., Liu, L., Shan, H., Lei, C.-L., Hui, D.S.C., et al. (2020). Clinical Characteristics of Coronavirus Disease 2019 in China. N. Engl. J. Med.
- Guérin, C., Reignier, J., Richard, J.-C., Beuret, P., Gacouin, A., Boulain, T., Mercier, E., Badet, M., Mercat, A., Baudin, O., et al. (2013). Prone positioning in severe acute respiratory distress syndrome. N. Engl. J. Med. 368, 2159–2168.
- Hardin, C.C., Malhotra, R., Petranovic, M., Klassen, S., Mihatov, N., and Oakley, D.H. (2019). Case 23-2019: A 52-Year-Old Man with Fever, Cough, and Hypoxemia. N. Engl. J. Med. 381, 359–369.
- Harris, R.S. (2005). Pressure-volume curves of the respiratory system. Respir. Care 50, 78–98; discussion 98–99.
- Manning, H.L., and Schwartzstein, R.M. (1995). Pathophysiology of dyspnea. N. Engl. J. Med. 333, 1547–1553.
- Mead, J., Takishima, T., and Leith, D. (1970). Stress distribution in lungs: a model of pulmonary elasticity. J. Appl. Physiol. 28, 596–608.
- Naeije, R., and Brimioulle, S. (2001). Physiology in medicine: importance of hypoxic pulmonary vasoconstriction in maintaining arterial oxygenation during acute respiratory failure. Crit. Care 5, 67–71.
- Swenson, E.R., and Bärtsch, P. (2012). High-altitude pulmonary edema. Compr. Physiol. 2, 2753–2773.
- Thompson, B.T., Chambers, R.C., and Liu, K.D. (2017). Acute Respiratory Distress Syndrome. N. Engl. J. Med. 377, 562–572.
- Tomashefski, J.F., Jr (2000). Pulmonary pathology of acute respiratory distress syndrome. Clin. Chest Med. 21, 435–466.
- Villar, J., Schultz, M.J., and Kacmarek, R.M. (2016). The LUNG SAFE: a biased presentation of the prevalence of ARDS! Crit. Care 20, 108.
- Wang, D., Hu, B., Hu, C., Zhu, F., Liu, X., Zhang, J., Wang, B., Xiang, H., Cheng, Z., Xiong, Y., et al. (2020). Clinical Characteristics of 138 Hospitalized Patients With 2019 Novel Coronavirus-Infected Pneumonia in Wuhan, China. JAMA.
- Ware, L.B., and Matthay, M.A. (2000). The acute respiratory distress syndrome. N. Engl. J. Med. 342, 1334–1349.
- Xu, Z., Shi, L., Wang, Y., Zhang, J., Huang, L., Zhang, C., Liu, S., Zhao, P., Liu, H., Zhu, L., et al. (2020). Pathological findings of COVID-19 associated with acute respiratory distress syndrome. Lancet Respir Med.
- Yang, X., Yu, Y., Xu, J., Shu, H., Xia, J. ’an, Liu, H., Wu, Y., Zhang, L., Yu, Z., Fang, M., et al. (2020). Clinical course and outcomes of critically ill patients with SARS-CoV-2 pneumonia in Wuhan, China: a single-centered, retrospective, observational study. Lancet Respir Med.
- Zhang, H., Zhou, P., Wei, Y., Yue, H., Wang, Y., Hu, M., Zhang, S., Cao, T., Yang, C., Li, M., et al. (2020). Histopathologic Changes and SARS-CoV-2 Immunostaining in the Lung of a Patient With COVID-19. Ann. Intern. Med.
View all COVID-19 updates
Learn about research in the Division of Pulmonary and Critical Care Medicine