Sedation in COVID-19
The FLARE Four
- ICU sedation should be minimized to the extent possible and protocols should avoid agents which lead to prolonged sedation such as benzodiazepines, as these can increase the rate of ICU related complications
- However, respiratory drive is increased in hypoxemic respiratory failure and this can lead to a perceived need for deep sedation in patients maintained on lung protective ventilation
- Preferred sedatives like propofol are also associated with complications such as hypertriglyceridemia and propofol infusion syndrome (PRIS) but severe side effects are fortunately rare
- Little data exists on safe de-escalation of sedation but patients who have been maintained on high doses of agents such as opiates or benzodiazepines likely require protocolized weaning
Many people are saying...I am using really high doses of sedation for my ventilated COVID-19 patients.
Subscribe to the latest updates from FLARE Advances in Motion
Introduction
Across the country, centers have reported increased sedation needs among intubated ICU patients during the COVID-19 epidemic. Tonight, we review the factors that may contribute to increased respiratory drive in ARDS as well as the evidence for adequate sedation in low tidal volume ventilation.
What Determines Respiratory Drive (and Thus Pushes Us to Reach for More Sedation in Ventilated Patients)
Under normal circumstances, central chemoreceptors regulate the ventilatory response to stabilize CO2. The following pathologic conditions can interfere with normal respiratory drive:
- Severe hypoxemia: peripheral chemoreceptors can enhance the ventilatory response to CO2 and thus increase the neural respiratory drive
- ARDS: lung inflammation and altered mechanics may be associated with alterations in neural respiratory drive. Vagal afferents from lung chemoreceptors increase respiratory rate and depth of breathing through a central reflex pathway
- Atelectasis: decreased inhibitory input from mechanoreceptors in atelectatic lung may further increase a patient’s respiratory drive
- Decreased lung and chest wall compliance: these can both alter the neuro-mechanical coupling between effort and diaphragmatic excursion
These circumstances lead to deviation from normal breathing pattern toward smaller achieved tidal volumes (VT) and tachypnea. The mechanical constraint on tidal volume leads to increased dyspnea for any given level of CO2. In extreme cases, in conjunction with elevated dead space and increased VD/VT, this may lead to hypercapnia. Hypercapnia then further stimulates breathing (Spinelli et al. 2020)
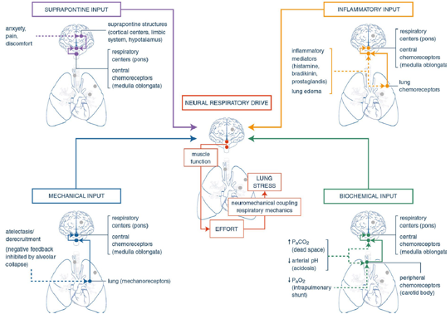
Figure 1
Key triggers of respiratory drive and anatomic targets where these triggers exert their effects (Spinelli et al. 2020).
Why Is Sedation So Difficult During Low Tidal Volume Ventilation for COVID-19 ARDS?
Evidence shows lower levels of sedation and non-benzodiazepine regimens may decrease duration of mechanical ventilation, decrease ICU days, and increase days free of delirium and coma (Shah, Girard, and Yende 2017; Shehabi et al. 2013). However, the need for patient ventilator synchrony in hypoxemic respiratory failure may lead to a perceived need for deeper sedation. Unfortunately, despite many of the landmark studies on ICU sedation containing significant numbers of patients with pneumonia or ARDS, they largely excluded patients requiring deeper sedation (see Table below) and so only offer limited information on the accuracy of this perception.
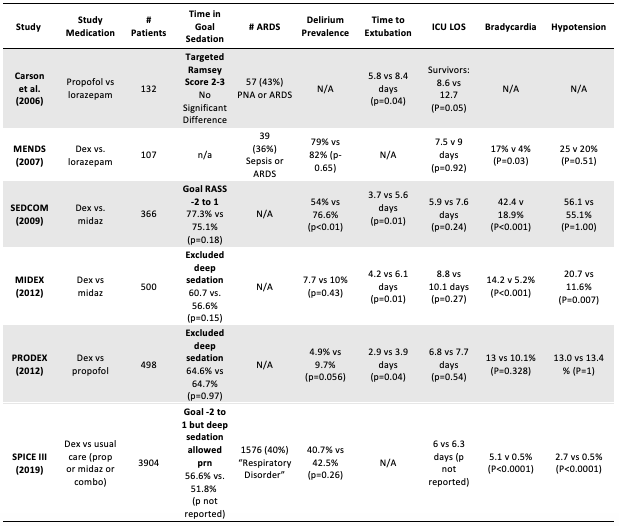
Figure 1
Adapted from: (Farina and Alaniz 2020).
Several small studies have attempted to evaluate the effect of low tidal volume ventilation (LTVV) on sedation needs, generally comparing the amount of sedation required in patients receiving tidal volumes of 6 mL/kg versus 12 mL/kg ideal body weight (Cheng et al. 2005; Mehta et al. 2014; Kahn et al. 2005). Due to small sample sizes, these trials are limited in their power to detect statistically significant differences between study groups. The majority of them report that LTVV did not result in increases in sedation use. This is an attractive, counterintuitive result, but methodological issues limit the enthusiasm with which these studies are interpreted. In addition to small sample size, issues include the fact that depth of sedation was not measured utilizing validated tools such as the Richmond Agitation Sedation Scale (RASS) (Sessler et al. 2002) and that these trials did not assess the role of ventilatory asynchrony leading to worsening ventilator induced lung injury.
Some additional insight may be gained from the ROSE PETAL trial, which evaluated the use of neuromuscular blockade (NMB) in ARDS. Patients with moderate to severe ARDS with a P:F <150mmHg and PEEP ≥ 8 cm H2O were randomized to receive 48 hours of NMB versus usual care (defined by a light sedation target of RASS 0 to -1 without continuous NMB). All patients received LTVV and a high PEEP strategy for up to 5 days after randomization; use of NMB for persistent pPlat > 30cm H2O was allowed. The investigators found no difference in in-hospital mortality, ventilator free days, or days out of the ICU between the two groups. However, there was a trend towards increased risk of serious cardiovascular events in the intervention group. In addition, despite a goal level of sedation defined as 0 to -1 by study investigators, supplemental material shows a deeper achieved level of sedation in the control group (average RASS of -2.3 to -2.7 over the first 48 hours) and 17% of control group patients required open label paralysis within the first 48 hours (National Heart, Lung, and Blood Institute PETAL Clinical Trials Network et al. 2019). Thus even in the control group - with no NMB - usual care resulted in significant sedation while targeting LTVV.
Much of what drives the increased sedation during lung protective ventilation is the desire to limit patient ventilator asynchrony. Asynchrony results when a patient on the ventilator makes spontaneous effort that has the potential to worsen lung injury. Double-triggering describes two consecutive inspirations without complete expiration and may be injurious because the delivered tidal volume is the sum of the two consecutive inspired tidal volumes. The impact of double triggering and other patient–ventilator asynchronies is increasingly recognized. Recent data from 50 ventilated patients suggest an association between increased incidence of asynchrony and higher mortality (Blanch et al. 2015).
Overall, patients on low tidal volume ventilation are perceived to need, and often receive, higher levels of sedation, even if the evidence for that practice is somewhat sparse.
My Patients on Propofol All Have High Triglycerides – Is This Propofol Infusion Syndrome(PRIS)?
Many centers preferentially use propofol (which is produced in a fatty emulsion) for sedation in order to avoid benzodiazepines and other agents that are associated with worse outcomes (Shehabi et al. 2013). Although several cases of propofol-associated hypertriglyceridemia and pancreatitis have been reported, information characterizing the frequency, severity, and response of clinicians to these sequelae is scarce (Devlin, Lau, and Tanios 2005). Devlin and colleagues describe an 18% incidence of hypertriglyceridemia (however, only 30% of patients receiving propofol actually had triglycerides measured, perhaps selecting for patients suspected to have high triglycerides levels). The propofol package labeling describes hypertriglyceridemia occurring in 3-10% of patients (Propofol Package Insert, n.d.).
Propofol infusion syndrome (PRIS), a feared complication of propofol, is most commonly associated with high dose and long duration use (Diedrich and Brown 2011). It is a diagnosis of exclusion and has had varying definitions over the years. A recent review of available literature suggested an updated definition: PRIS may occur in critically ill patients receiving propofol infusions, typically either high dose (>5 mg/kg/hr [83 mcg/kg/min]) or of long duration (>48 h), and is characterized by one or more of otherwise unexplained metabolic acidosis, rhabdomyolysis, or ECG changes, with or without acute kidney injury, cardiac failure, fever, or elevated potassium, lipids, liver enzymes, or lactate (Hemphill et al. 2019). PRIS is thought to be due to mitochondrial toxicity via multiple proposed mechanisms such as interaction with Coenzyme Q of cytochrome C and/or inhibition of fatty acid oxidation resulting in reduced ATP production (Vanlander et al. 2015; Hemphill et al. 2019). PRIS incidence has been reported as ≤ 1% in adults but may be higher in patients with pre-existing mitochondrial disease, and notably, may not be associated with hypertriglyceridemia (Roberts et al. 2009). An additional review of all published case reports of PRIS found hyperlipidemia present in only 22% of adult PRIS cases (Hemphill et al. 2019).
Based on this low incidence, triglycerides and CK elevations in critically ill COVID-19 patients likely reflect an underlying inflammatory state rather than PRIS; however, clinicians should monitor and consider PRIS diagnosis in cases of sudden and otherwise unexplained cardiovascular compromise.
Why Won’t My Patient Wake up After I Stop Sedation?
Critically ill patients have substantially altered pharmacokinetic (PK) and pharmacodynamic (PD) parameters as a result of hemodynamic instability, fluid resuscitation, altered protein binding, and organ dysfunction from severe systemic illness (Tse et al. 2018).
For many anesthetic and sedative agents, accumulation in fat, muscle, and viscera during continuous infusion leads to changes in elimination. In pharmacokinetics, the elimination half life is the rate of drug elimination within a one-compartment model (i.e. for drugs that do not accumulate in peripheral tissue), and may not accurately predict the duration of effects for drugs with multi-compartment models. The context-sensitive half-time is the time required for blood or plasma concentrations of a drug to decrease by 50% after discontinuation of drug administration. The context-sensitive half-time often cannot be predicted by the elimination half-life and duration of infusion, and can help explain the duration of action of a drug after stopping an infusion.
Consider fentanyl: at baseline, fentanyl administered as an intravenous bolus would have an expected duration of action of 30-60 minutes. After a 6 hour infusion, the half life increases to 200 minutes, and further increases to 300 minutes after 12 hours of infusion. In patients with ARDS secondary to COVID-19, the time on mechanical ventilation (often prolonged LTVV) is reported to be in the range of 1-2 weeks (Ziehr et al. 2020). This can lead to prolonged anesthetic and sedative infusions and make the pharmacokinetics of medications highly unpredictable. With prolonged use, it is likely that there will be prolonged sedation once this agent is discontinued (see Figure 2). Other sedative and anesthetic medications with large volumes of distribution and high lipophilicity may also have these effects (Hughes, Glass, and Jacobs 1992; Spina and Ensom 2007).
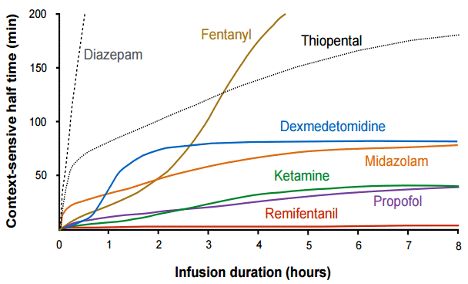
Figure 2
Context-sensitive half-time of various sedative agents. Context-sensitive half-time is the time required for blood or plasma concentrations of a drug to decrease by 50% after discontinuation of drug administration. This measure takes into account duration of infusion and multi-compartment distribution of a drug (fat, muscle, and viscera, for example) (Hughes, Glass, and Jacobs 1992).
Midazolam is another agent whose pharmacokinetics change significantly with prolonged continuous infusion. These changes include depot formation in peripheral adipose tissues which results in prolonged duration of action. Obese patients, by nature of their excess adipose tissue and thus accumulation of drug and metabolite, appear to be at heightened risk for prolonged sedation; clinical recovery in these patients can be delayed for hours to days (Spina and Ensom 2007). Finally, increases in the volume of distribution in the critically ill can result in a prolonged half life and decreased clearance of the medication, leading to accumulation over time. Patients with high inflammatory states or renal failure may have reduced the enzyme activity of CYP3A4, leading to accumulation of parent drug. The active metabolite, alpha1-hydroxymidazolam, is 20% less potent than the parent compound, with a half-life of approximately 0.8–1 hour in patients with normal renal function, but increases to >25 hours in patients with acute kidney impairment (Spina and Ensom 2007).
In addition to slow awakening due to prolonged infusions of sedative agents, critically-ill COVID-19 patients, like all critically ill patients, are vulnerable to the delirium (particularly hypoactive) and cognitive impairment experienced by survivors of critical illness (Salluh et al. 2015; Peterson et al. 2006). ARDS survivors have been reported to experience a high prevalence of cognitive impairment: 70-100% at hospital discharge, 46-80% at 1 year, and 20% at 5 years (Sasannejad, Ely, and Lahiri 2019). A combination of non-modifiable clinical risk factors (presenting brain injury, pre-existing dementia, history of neurological injury), pathophysiological events (blood brain barrier dysfunction, inflammation and cytokine circulation, hypoxemia, hypoperfusion, amyloid deposition), and modifiable clinical risk factors (delirium, sedation, mechanical ventilation, hemodynamic instability) create an aggregate risk for short and long-term cognitive impairment after acute illness.
In summary, prolonged use of these agents in a critically ill patient population may lead to unpredictable pharmacokinetics and prolonged duration of action. Delirium and cognitive impairment are also of high prevalence in ARDS patients and may contribute to a prolonged time to awaken.
How Do I Wean My COVID-19 Patient From Sedation?
The primary concern with rapid discontinuation of sedative medication is fear of inducing an iatrogenic withdrawal syndrome, particularly with use of benzodiazepines and opiates. Risk factors for iatrogenic withdrawal syndrome have been primarily reported in pediatric ICU data and have been identified as hours of midazolam use, number of days of sedation, mean daily opioid dose, baseline cognitive impairment, and patients receiving three or more sedative classes. In adult patients, one study identified the total dose of opioids as a risk factor for iatrogenic withdrawal (Chiu et al. 2017). In the sparse adult literature, iatrogenic opioid withdrawal has been described at rates of 16.8-32.1%, but with significant heterogeneity in the definitions of opioid withdrawal, methods, and patient populations between studies (Chiu et al. 2017).
The majority of evidence for weaning strategies comes from the pediatric literature. Aside from the obvious differences in patient population and concerns regarding applicability to an adult patient population, the assessment of withdrawal in these patients is also different. There are currently two validated pediatric critically ill opioid assessment tools: the Withdrawal Assessment Tool-Version 1 (WAT-1) and Sophia Observation Withdrawal Symptoms Scale (SOS). There are no currently validated assessment tools for the adult critically ill patient population to detect iatrogenic withdrawal. However, clinical opiate withdrawal scale (COWS) has been utilized in non-critically ill adult patients with opioid dependence (Wesson and Ling 2003). A recent meta-analysis of pediatric opioid and benzodiazepine weaning literature found that utilization of a standardized protocol had lower rates of withdrawal, ranging from 5-22% as compared to 24-80% to non-standardized approaches or those with low protocol adherence (Fenn and Plake 2017).
Dexmedetomidine withdrawal syndrome, characterized by sympathetic overactivity, has been described in prior case reports and a recent review of pediatric and adult patients (Glaess, Attridge, and Christina Gutierrez 2020). Duration of dexmedetomidine infusion prior to the development of a withdrawal syndrome shows wide inter-patient variability, including as little as 3 days.
Rate of Weaning of Infusions and Use of Adjunctive Therapies
The primary method of weaning from sedative infusions without precipitating a withdrawal syndrome is a slow and steady decrease in the rate of infusion (i.e. by 25% or so per day). This can result in a prolonged wean and there are some data (see below) supporting the addition of longer acting medications (e.g. methadone for opiates, lorazepam for midazolam, and clonidine in the case of dexmedetomidine) in order to decrease infusion rates more rapidly.
Benzodiazepines
Lorazepam has been described in pediatric literature as an option to facilitate weaning from midazolam infusions, but outcomes data are scarce and benzodiazepine weaning protocols were often evaluated with concomitant opioid weaning strategies, making it difficult to differentiate between different withdrawal syndromes. Phenobarbital use has been described as an adjunctive agent in neonatal abstinence syndrome (Raffaeli et al. 2017) and in alcohol withdrawal syndromes, but has not yet been described for the treatment of iatrogenic benzodiazepine syndrome.
Opioids
In a study of critically ill adult patients, Wanzuita et al. found that mechanical ventilation was significantly shorter in a methadone-treated group compared to control (HR=2.06; 95%CI 1.17-3.63, P<0.004) (Wanzuita et al. 2012). Al-Qadheeb and colleagues similarly found that time to fentanyl discontinuation was shorter in the methadone-treated group versus control (4.5 vs 7 days; p= 0.002) in a cohort of mechanically ventilated patients without history of opioid dependence in an adult medical ICU (Al-Qadheeb et al. 2012). Despite these promising results, optimal dosing and duration of a methadone wean are yet to be determined.
Dexmedetomidine
Case reports describing the use of clonidine for prevention of dexmedetomidine withdrawal syndromes have significant heterogeneity in the clonidine intervention; with starting doses reported from 0.1mg q8h to 0.5mg q6h. Across studies, the use of clonidine showed successful dexmedetomidine discontinuation in 65-75% of patients within 24-48 hours (Glaess, Attridge, and Christina Gutierrez 2020). Transitioning to a non-parenteral medication such as clonidine has the potential to accelerate a patient’s course by decreasing the need for ICU level care. Additionally, clonidine is an established adjunctive therapy for withdrawal symptom management in other settings and it may attenuate withdrawal symptoms from other common adjunctive agents for sedation management (ie, opioids and benzodiazepines).
In summary, data regarding optimal sedation weaning strategies in adult ICU patients are scarce. Weaning protocols utilizing longer-acting oral forms of intravenous infusions, such as methadone, lorazepam and clonidine, may facilitate more rapid discontinuation.
Summary
Patients with respiratory failure from COVID-19 have been characterized as “difficult to sedate,” which is likely related to the underlying pathophysiology of ARDS, further compounded by alterations in drug pharmacokinetics and pharmacodynamics occurring in the critically ill patient undergoing prolonged mechanical ventilation. To achieve satisfactory low tidal volume ventilation, patients may need substantial sedation, which may precipitate challenging sedation weaning, including slow awakening, delirium, and cognitive impairment. Care should be taken to carefully taper sedative medications. Given the complexity of the multi-modal sedation strategies required in ARDS patients, we recommend consultation with specialty critical care pharmacists when available.
References:
- Al-Qadheeb, Nada S., Russel J. Roberts, Ryan Griffin, Erik Garpestad, Robin Ruthazer, and John W. Devlin. 2012. “Impact of Enteral Methadone on the Ability to Wean off Continuously Infused Opioids in Critically Ill, Mechanically Ventilated Adults: A Case-Control Study.” The Annals of Pharmacotherapy 46 (9): 1160–66.
- Blanch, Lluís, Ana Villagra, Bernat Sales, Jaume Montanya, Umberto Lucangelo, Manel Luján, Oscar García-Esquirol, et al. 2015. “Asynchronies during Mechanical Ventilation Are Associated with Mortality.” Intensive Care Medicine. https://doi.org/10.1007/s00134-015-3692-6.
- Cheng, Ivan W., Mark D. Eisner, B. Taylor Thompson, Lorraine B. Ware, and Michael A. Matthay. 2005. “Acute Effects of Tidal Volume Strategy on Hemodynamics, Fluid Balance, and Sedation in Acute Lung Injury*.” Critical Care Medicine. https://doi.org/10.1097/01.ccm.0000149836.76063.71.
- Chiu, Ada W., Sofia Contreras, Sangeeta Mehta, Jennifer Korman, Marc M. Perreault, David R. Williamson, and Lisa D. Burry. 2017. “Iatrogenic Opioid Withdrawal in Critically Ill Patients: A Review of Assessment Tools and Management.” The Annals of Pharmacotherapy 51 (12): 1099–1111.
- Devlin, John W., Adah K. Lau, and Maged A. Tanios. 2005. “Propofol-Associated Hypertriglyceridemia and Pancreatitis in the Intensive Care Unit: An Analysis of Frequency and Risk Factors.” Pharmacotherapy. https://doi.org/10.1592/phco.2005.25.10.1348.
- Diedrich, Daniel A., and Daniel R. Brown. 2011. “Analytic Reviews: Propofol Infusion Syndrome in the ICU.” Journal of Intensive Care Medicine 26 (2): 59–72.
- Farina, Nicholas, and Cesar Alaniz. 2020. “Reconsidering Dexmedetomidine for Sedation in the Critically Ill: Implications of the SPICE III Trial.” The Annals of Pharmacotherapy 54 (5): 504–8.
- Fenn, Norman E., and Kimberly S. Plake. 2017. “Opioid and Benzodiazepine Weaning in Pediatric Patients: Review of Current Literature.” Pharmacotherapy: The Journal of Human Pharmacology and Drug Therapy. https://doi.org/10.1002/phar.2026.
- Glaess, Shelley S., Rebecca L. Attridge, and G. Christina Gutierrez. 2020. “Clonidine as a Strategy for Discontinuing Dexmedetomidine Sedation in Critically Ill Patients: A Narrative Review.” American Journal of Health-System Pharmacy. https://doi.org/10.1093/ajhp/zxaa013.
- Hemphill, Scott, Luke McMenamin, Mark C. Bellamy, and Philip M. Hopkins. 2019. “Propofol Infusion Syndrome: A Structured Literature Review and Analysis of Published Case Reports.” British Journal of Anaesthesia 122 (4): 448–59.
- Hughes, M. A., P. S. Glass, and J. R. Jacobs. 1992. “Context-Sensitive Half-Time in Multicompartment Pharmacokinetic Models for Intravenous Anesthetic Drugs.” Anesthesiology 76 (3): 334–41.
- Kahn, Jeremy M., Liane Andersson, Veena Karir, Nayak L. Polissar, Margaret J. Neff, and Gordon D. Rubenfeld. 2005. “Low Tidal Volume Ventilation Does Not Increase Sedation Use in Patients with Acute Lung Injury*.” Critical Care Medicine. https://doi.org/10.1097/01.ccm.0000157786.41506.24.
- Mehta, Sangeeta, for the Lung Open Ventilation Study Investigators, Deborah J. Cook, Yoanna Skrobik, John Muscedere, Claudio M. Martin, Thomas E. Stewart, Lisa D. Burry, Qi Zhou, and Maureen Meade. 2014. “A Ventilator Strategy Combining Low Tidal Volume Ventilation, Recruitment Maneuvers, and High Positive End-Expiratory Pressure Does Not Increase Sedative, Opioid, or Neuromuscular Blocker Use in Adults with Acute Respiratory Distress Syndrome and May Improve Patient Comfort.” Annals of Intensive Care. https://doi.org/10.1186/s13613-014-0033-9.
- National Heart, Lung, and Blood Institute PETAL Clinical Trials Network, Marc Moss, David T. Huang, Roy G. Brower, Niall D. Ferguson, Adit A. Ginde, M. N. Gong, et al. 2019. “Early Neuromuscular Blockade in the Acute Respiratory Distress Syndrome.” The New England Journal of Medicine 380 (21): 1997–2008.
- Peterson, Josh F., Brenda T. Pun, Robert S. Dittus, Jason W. W. Thomason, James C. Jackson, Ayumi K. Shintani, and E. Wesley Ely. 2006. “Delirium and Its Motoric Subtypes: A Study of 614 Critically Ill Patients.” Journal of the American Geriatrics Society 54 (3): 479–84.
- Propofol Package Insert. n.d. “Propofol [Package Insert]. Lake Zurich, IL: Fresenius Kabi; 2014.”
- Raffaeli, Genny, Giacomo Cavallaro, Karel Allegaert, Enno Diederik Wildschut, Monica Fumagalli, Massimo Agosti, Dick Tibboel, and Fabio Mosca. 2017. “Neonatal Abstinence Syndrome: Update on Diagnostic and Therapeutic Strategies.” Pharmacotherapy 37 (7): 814–23.
- Roberts, Russel J., Jeffrey F. Barletta, Jeffrey J. Fong, Greg Schumaker, Philip J. Kuper, Stella Papadopoulos, Dinesh Yogaratnam, et al. 2009. “Incidence of Propofol-Related Infusion Syndrome in Critically Ill Adults: A Prospective, Multicenter Study.” Critical Care. https://doi.org/10.1186/cc8145.
- Salluh, Jorge I. F., Han Wang, Eric B. Schneider, Neeraja Nagaraja, Gayane Yenokyan, Abdulla Damluji, Rodrigo B. Serafim, and Robert D. Stevens. 2015. “Outcome of Delirium in Critically Ill Patients: Systematic Review and Meta-Analysis.” BMJ 350 (June): h2538.
- Sasannejad, Cina, E. Wesley Ely, and Shouri Lahiri. 2019. “Long-Term Cognitive Impairment after Acute Respiratory Distress Syndrome: A Review of Clinical Impact and Pathophysiological Mechanisms.” Critical Care / the Society of Critical Care Medicine 23 (1): 352.
- Sessler, Curtis N., Mark S. Gosnell, Mary Jo Grap, Gretchen M. Brophy, Pam V. O’Neal, Kimberly A. Keane, Eljim P. Tesoro, and R. K. Elswick. 2002. “The Richmond Agitation–Sedation Scale.” American Journal of Respiratory and Critical Care Medicine 166 (10): 1338–44.
- Shah, Faraaz Ali, Timothy D. Girard, and Sachin Yende. 2017. “Limiting Sedation for Patients with Acute Respiratory Distress Syndrome – Time to Wake up.” Current Opinion in Critical Care. https://doi.org/10.1097/mcc.0000000000000382.
- Shehabi, Yahya, The Sedation Practice in Intensive Care Evaluation (SPICE) Study Group investigators, Lucy Chan, Suhaini Kadiman, Anita Alias, Wan Nasrudin Ismail, Mohd Ali Ismail, et al. 2013. “Sedation Depth and Long-Term Mortality in Mechanically Ventilated Critically Ill Adults: A Prospective Longitudinal Multicentre Cohort Study.” Intensive Care Medicine. https://doi.org/10.1007/s00134-013-2830-2.
- Spina, Sean P., and Mary H. H. Ensom. 2007. “Clinical Pharmacokinetic Monitoring of Midazolam in Critically Ill Patients.” Pharmacotherapy 27 (3): 389–98.
- Spinelli, Elena, Tommaso Mauri, Jeremy R. Beitler, Antonio Pesenti, and Daniel Brodie. 2020. “Respiratory Drive in the Acute Respiratory Distress Syndrome: Pathophysiology, Monitoring, and Therapeutic Interventions.” Intensive Care Medicine. https://doi.org/10.1007/s00134-020-05942-6.
- Tse, Andrew H. W., Lowell Ling, Anna Lee, and Gavin M. Joynt. 2018. “Altered Pharmacokinetics in Prolonged Infusions of Sedatives and Analgesics Among Adult Critically Ill Patients: A Systematic Review.” Clinical Therapeutics 40 (9): 1598–1615.e2.
- Vanlander, Arnaud Vincent, Juergen Guenther Okun, Annick de Jaeger, Joél Smet, Elien De Latter, Boel De Paepe, Georges Dacremont, et al. 2015. “Possible Pathogenic Mechanism of Propofol Infusion Syndrome Involves Coenzyme Q.” Anesthesiology 122 (2): 343–52.
- Wanzuita, Raquel, Luiz F. Poli-de-Figueiredo, Felipe Pfuetzenreiter, Alexandre Cavalcanti, and Glauco Westphal. 2012. “Replacement of Fentanyl Infusion by Enteral Methadone Decreases the Weaning Time from Mechanical Ventilation: A Randomized Controlled Trial.” Critical Care. https://doi.org/10.1186/cc11250.
- Wesson, Donald R., and Walter Ling. 2003. “The Clinical Opiate Withdrawal Scale (COWS).” Journal of Psychoactive Drugs 35 (2): 253–59.
- Ziehr, David R., Jehan Alladina, Camille R. Petri, Jason H. Maley, Ari Moskowitz, Benjamin D. Medoff, Kathryn A. Hibbert, B. Taylor Thompson, and C. Corey Hardin. 2020. “Respiratory Pathophysiology of Mechanically Ventilated Patients with COVID-19: A Cohort Study.” American Journal of Respiratory and Critical Care Medicine, April. https://doi.org/10.1164/rccm.202004-1163LE.
Learn more about research in the Division of Pulmonary and Critical Care Medicine
View all COVID-19 updates