ECMO for COVID-19 (Part I)
The FLARE Four
- Veno-venous extracorporeal membrane oxygenation (VV ECMO) is a form of pulmonary bypass that allows oxygenation to occur via an external membrane
- ECMO use has rapidly expanded in recent years, spurred on by the last respiratory virus pandemic - the 2009 H1N1 influenza outbreak - and the completion of two large randomized controlled trials
- Use of ECMO has been advocated for based on its ability to improve gas exchange and potentially prevent or reduce ventilator-induced lung injury
- Trial data and guidelines support the use of ECMO as rescue therapy in severe ARDS after failure of conventional ARDS management
Many people are saying...FLARE should discuss ECMO.
Subscribe to the latest updates from FLARE Advances in Motion
Introduction
Extracorporeal membrane oxygen oxygenation (ECMO) is an option to support refractory respiratory failure which has seen dramatic increases in use in recent years. The first NIH sponsored trial of ECMO for respiratory failure was performed in 1979 (Zapol et al., 1979). At that time, no difference in outcome between the ECMO and conventional therapy groups was noted - both reporting a ~90% mortality. During the 2009 H1N1 pandemic, improved technology led to an expansion in ECMO use (Davies et al., 2009) with a rapid rise in both the number of patients treated with ECMO and the number of centers able to provide it. ECMO has subsequently been effectively used for non-SARS-CoV-2 coronavirus infections such as MERS (Alshahrani et al., 2018). As of 5/8, the Extracorporeal Life Support Organization (ELSO), an international society that collects data on ECMO, reports over 750 uses of ECMO for COVID-19 worldwide (Table 1). This is certainly an underestimate given reporting delays, lack of reporting by non-ELSO chapters, and exclusion of centers that have fewer than 5 cases.
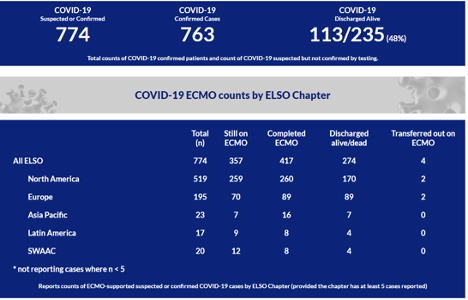
Table 1
ELSO report of ECMO use in COVID worldwide, as of 5/8 (ELSO website).
In this two part FLARE, we will explore the use of ECMO in ARDS generally, and in COVID-19-related ARDS in particular. Tonight, we begin by examining the use of ECMO in ARDS.
How Does ECMO Work?
VV ECMO bypasses the need for pulmonary gas exchange by diverting venous blood through an external membrane. A fraction of a patient’s cardiac output is removed from the vena cava before reaching the right atrium, pumped and circulated through a membrane where gas exchange occurs. Gas exchange in the ECMO membrane is conceptually similar to gas exchange in a healthy lung - fresh gas with a high fraction of O2 and no CO2 is swept across one side of the membrane and blood flows across the other - gas exchange occurs by diffusion. Oxygenated blood is then delivered back into the vena cava where it mixes with central venous blood and proceeds to the lungs (Figure 1). Depending on the function of the patient’s lungs and and ventilator settings, the patient’s lungs may or may not contribute to further oxygenation of the mixed venous blood as it flows through the pulmonary circulation. Oxygenation of blood via VV ECMO depends on the fraction of delivered oxygen (referred to as FdO2) in the gas sweeping across the membrane and the fraction of cardiac output that is circulated through the ECMO circuit. Increasing blood flow through the membrane by adjusting the pump, or, perhaps counterintuitively, decreasing the patient’s cardiac output (often via beta blockade), may improve oxygenation. Carbon dioxide removal can be enhanced by increasing “sweep”, or the rate of gas flow across the membrane (Figure 1).
Veno-arterial (VA) ECMO, not within the scope of tonight’s FLARE, also provides circulatory support, effectively providing cardiopulmonary bypass.
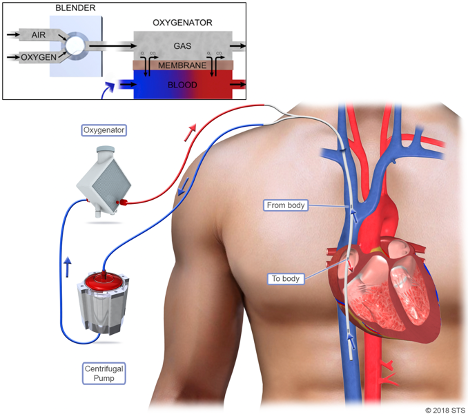
Figure 1
VV ECMO circuit. Blood is removed from the superior vena cava (SVC) and inferior vena cava (IVC), pumped through an oxygenator (aka “membrane”), and returned to the right atrium. A single cannula placed in the right internal jugular (RIJ) vein is shown. Alternatively, blood can be drained from the IVC via a femoral vein cannula, and returned to the SVC via a RIJ cannula. (Image adapted from STS 2008 and Abrams and Brodie, 2017.)
What Do Randomized Controlled Trials Tell Us About ECMO in ARDS?
Prior to the late 2000s, there were only two randomized controlled trials that looked at ECMO in ARDS: one published in 1979 (Zapol et al., 1979) and the other in 1994 (Morris et al., 1994). Neither of these studies demonstrated a mortality benefit in the ECMO arm, but these results are difficult to apply currently given changes in case selection, circuit design, and ventilation strategies in modern ECMO. In 2009, the Conventional ventilation or ECMO for Severe Adult Respiratory failure (CESAR) trial (Peek et al., 2009) randomized 180 patients to either conventional management or consideration for support with ECMO. They found that the ECMO arm had higher survival without disability at 6 months compared to control (75% vs. 47%, p = 0.03). However, this study was hampered by the fact that only 70% of those in the control arm were treated with a low tidal volume strategy while the ECMO arm employed lung protective ventilation in 93% of cases (p < 0.0001). Further, only 42% of the control arm received pronation, and 25% of those assigned to the ECMO arm were never actually cannulated. Due to these issues, it was not possible to conclude from the CESAR trial whether ECMO was superior to evidence-based ARDS management including low tidal volume ventilation and prone positioning.
EOLIA, the largest and most recent RCT for ECMO in ARDS, was published in 2018 and attempted to correct the limitations of CESAR (Combes et al., 2018). Two-hundred forty-nine patients were randomized to either ECMO or conventional therapy with potential to cross-over into the ECMO arm if needed. Unlike in CESAR, the mean tidal volumes for both the control and treatment arm were 6.0 ml/kg and 6.1 ml/kg, respectively, and 62% of patients in the conventional management group were pronated. At 60 days, mortality in the ECMO group was 35%, compared to 46% in the control group, but this did not reach statistical significance (Figure 2). There were significantly higher rates of bleeding leading to transfusion in the ECMO arm (46%) versus the control arm (28%), but no difference in massive bleeding.
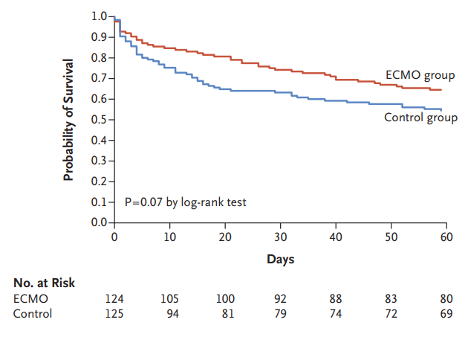
Figure 2
Kaplan-Meier survival curves for the ECMO and control groups in EOLIA (Combes et al., 2018).
An important feature of EOLIA is that 28% of patients in the control arm crossed over and received ECMO, but were analyzed based on their original randomization assignment. This meant that EOLIA was effectively a trial of ECMO as a rescue therapy versus upfront ECMO. A post-hoc bayesian analysis of the EOLIA trial (Goligher et al., 2018) suggested that ECMO is likely to reduce mortality across a range of prior probabilities. Many of the EOLIA patients who crossed over into the ECMO arm were quite sick at the time of crossover - some even initiated ECMO during advanced cardiac life support. In other words, if EOLIA was effectively a trial of upfront ECMO versus standard management with ECMO as rescue, it was with ECMO as late rescue. One might reasonably expect that real world use of rescue ECMO would result in earlier ECMO than seen in the EOLIA control arm. Therefore an even smaller difference in 60-day mortality could be seen between early ECMO and ECMO as early/real world rescue. Thus, the early use of ECMO in patients with severe ARDS lacks sufficient evidence to recommend it, but current evidence does support the role of ECMO as rescue therapy once conventional therapies for ARDS have failed.
Does ECMO Provide Benefits Other Than Improved Oxygenation?
Aside from improved oxygenation, ECMO may enhance lung protection, given the ability to use ultra-low tidal volumes while providing gas exchange via the ECMO circuit. The use of ECMO in support of lung rest ventilation ((Gattinoni et al., 1986) -- yes, that Gattinoni!) predates even ARDSNet publications (Acute Respiratory Distress Syndrome Network et al., 2000) demonstrating the benefit of low tidal volume ventilation. This was more recently demonstrated in a porcine ARDS model of near-apneic ventilation utilizing ECMO which achieved very low driving pressures when compared to both non-protective and conventional protective ventilatory strategies over a 24 hour period (Araos et al., 2019) (Figure 3).
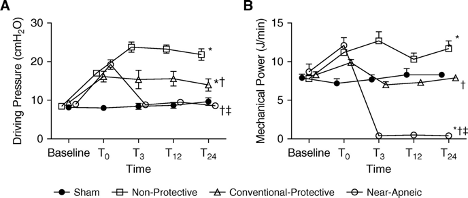
Figure 3
Near-apneic ventilation results in lower mechanical power and driving pressures versus conventional lung protective ventilation (Araos et al., 2019).
In this model, the resulting decrease in stress led to a noticeable decrease in histological damage, as measured by degree of diffuse alveolar damage noted on H&E staining (Figure 4, left image), as well as a decrease in staining for α-smooth muscle actin (α-SMA), a surrogate for myofibroblasts (Figure 4, right image).
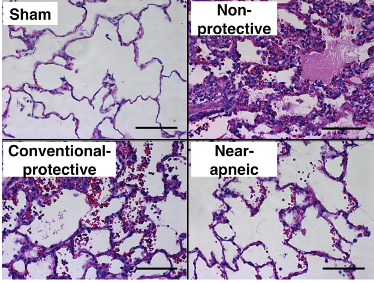
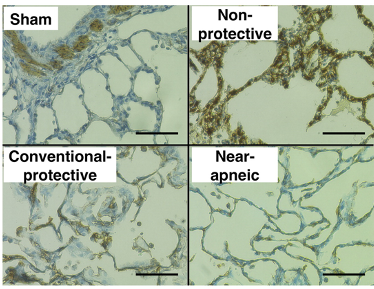
Figure 4
Histologic assessment of lung injury (left) and myofibroblasts (right). Left: Representative images of lung histology for each study group (hematoxylin and eosin, scale bar = 100 μm). Images from nonprotective and conventional protective groups presented diffuse alveolar damage with alveolar edema, hemorrhage, hyaline membranes, and inflammatory cells in the interstitium and alveolar spaces.
Bottom: Immunohistochemical staining of α-SMA (α-smooth muscle actin) protein as a surrogate for myofibroblasts in lung tissue preparations (scale bar = 50 μm). (Adapted from Araos et al., 2019.)
A similar attempt to evaluate the feasibility of ultra-low tidal volume (4 ml/kg) ventilation in humans without adjunct use of ECMO had mixed results - with 2/35 (6%) subjects developing acute cor pulmonale, 11/35 (32%) developing severe acidosis with pH < 7.15 and mortality was 41% (Richard et al., 2019). While ECMO clearly facilitates even lower plateau pressures and tidal volume than standard LTVV (Rozencwajg et al., 2019), there is currently no clinical evidence that combined use of ECMO with ultra-protective ventilation improves outcomes.
What Role Could VV ECMO Play in a Respiratory Virus Pandemic?
In 2009, the WHO declared a global flu pandemic (CDC 2020) due to influenza A H1N1. With the resulting increase in cases of severe ARDS, particularly among young patients (WHO 2011), came a renewal of interest in ECMO for the management of severe ARDS. By November 2009, the first multicenter study on the use of ECMO in H1N1, ANZ ECMO, was published (Davies et al., 2009). This observational study included 68 patients from Australia and New Zealand who were supported with ECMO across 15 specialized intensive care units. Criteria for cannulation were not provided, and likely varied greatly given the retrospective nature of the study and involvement of multiple centers. The median age was 34.4 (IQR 26.6-43.1) and the most common comorbidities were obesity (50%), asthma (15%), and diabetes mellitus (9%). Interestingly, similar characteristics are seen in those who have developed ARDS from COVID-19 (Bhatraju et al., 2020; Wu et al., 2020; Ziehr et al., 2020). The median lowest P:F ratio was 56 (IQR 48-63), indicating that selected patients had severe impairments in gas exchange. Survival at ICU discharge was 71%, while 9% of those studied remained in the unit at the end of the observation period. Cause of death was most frequently intracranial hemorrhage (43%), followed by hemorrhage and intractable respiratory failure, both at 29%.
These data are encouraging, but are muddled by the fact that this study preceded key innovations in non-ECMO care - particularly widespread implementation of proning that occurred after publication of the PROSEVA trial (Guérin et al., 2013). While rescue therapies like recruitment maneuvers (67%) and nitric oxide (32%) were employed, only 20% of patients were pronated. One can speculate that if only those who failed a trial of proning (and hence were sicker) were cannulated, perhaps the mortality rate would have been higher than 20%.
A French cohort study of 123 patients with H1N1 ARDS who received ECMO was later published. Fifty-two patients were matched with a non-ECMO control group using propensity scoring. This comparison found no difference in mortality or length of ICU stay (Pham et al., 2013). Overall mortality rate for those who received ECMO was 36%. However, those who could not undergo matching were younger (mean age 38 vs. 45, p < 0.01) and had significantly lower mortality (22% vs. 50%, p < 0.01) despite having significantly lower P:F ratios (54 vs. 70, p < 0.01). This suggests that younger age may portend prognostic benefit for those with ARDS secondary to viral pneumonia, although this has yet to be supported by prospective evidence.
What Evidence Do We Have for VV ECMO in Other Coronavirus Infections?
We have experience with ARDS caused by another type of coronavirus - MERS-CoV – which causes the Middle East Respiratory Syndrome (MERS). Seventeen patients with MERS-associated ARDS who received ECMO were compared to a group of 18 patients who met criteria for ECMO but received conventional therapy due to the unavailability of ECMO at the time (Alshahrani et al., 2018). The ECMO group had lower in-hospital mortality (65% vs. 100%, p = 0.02; see Figure 5) and similar hospital length of stay (41 vs. 31 days, p = 0.421).
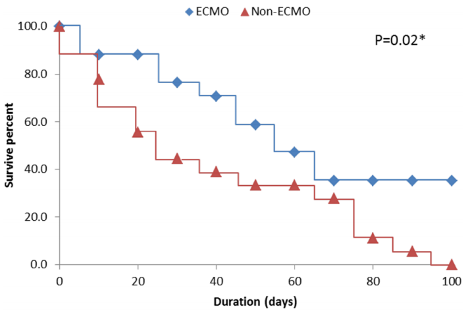
Figure 5
Kaplan-Meier survival curves for the ECMO and non-ECMO treatment groups in 18 patients with MERS-associated ARDS (Alshahrani et al., 2018).
While this shows signs of promise for ECMO in coronavirus-related ARDS, the sample size is far too small to draw any meaningful conclusions. Further it was reported that none of the patients received prone ventilation, which could have reduced mortality in the control arm. Despite the virologic link with MERS, it is difficult to rely on these data to inform decisions regarding ECMO in COVID-19.
Conclusions
Since the first uses of ECMO, the technology has undergone rapid development and has become more widely available. In response to the 2009 H1N1 pandemic, there has been a resurgence of interest in ECMO, spurred on by the completion of two large randomized controlled trials. The benefits of ECMO include improved blood oxygenation and avoiding ventilator-induced lung injury, and ECMO is now an established rescue therapy in ARDS. In tomorrow’s FLARE, we will explore the use of ECMO in ARDS related to COVID-19.
References:
- Abrams, D., and Brodie, D. (2017). Extracorporeal Membrane Oxygenation for Adult Respiratory Failure: 2017 Update. Chest 152, 639–649.
- Acute Respiratory Distress Syndrome Network, Brower, R.G., Matthay, M.A., Morris, A., Schoenfeld, D., Thompson, B.T., and Wheeler, A. (2000). Ventilation with lower tidal volumes as compared with traditional tidal volumes for acute lung injury and the acute respiratory distress syndrome. N. Engl. J. Med. 342, 1301–1308.
- Alshahrani, M.S., Sindi, A., Alshamsi, F., Al-Omari, A., El Tahan, M., Alahmadi, B., Zein, A., Khatani, N., Al-Hameed, F., Alamri, S., et al. (2018). Extracorporeal membrane oxygenation for severe Middle East respiratory syndrome coronavirus. Ann. Intensive Care 8, 3.
- Araos, J., Alegria, L., Garcia, P., Cruces, P., Soto, D., Erranz, B., Amthauer, M., Salomon, T., Medina, T., Rodriguez, F., et al. (2019). Near-Apneic Ventilation Decreases Lung Injury and Fibroproliferation in an Acute Respiratory Distress Syndrome Model with Extracorporeal Membrane Oxygenation. Am. J. Respir. Crit. Care Med. 199, 603–612.
- Bhatraju, P.K., Ghassemieh, B.J., Nichols, M., Kim, R., Jerome, K.R., Nalla, A.K., Greninger, A.L., Pipavath, S., Wurfel, M.M., Evans, L., et al. (2020). Covid-19 in Critically Ill Patients in the Seattle Region - Case Series. N. Engl. J. Med.
- CDC (2020). 2009 H1N1 Flu Pandemic Timeline.
- Combes, A., Hajage, D., Capellier, G., Demoule, A., Lavoué, S., Guervilly, C., Da Silva, D., Zafrani, L., Tirot, P., Veber, B., et al. (2018). Extracorporeal Membrane Oxygenation for Severe Acute Respiratory Distress Syndrome. N. Engl. J. Med. 378, 1965–1975.
- Davies, A., Jones, D., Bailey, M., Beca, J., Bellomo, R., Blackwell, N., Forrest, P., Gattas, D., Granger, E., Herkes, R., et al. (2009). Extracorporeal Membrane Oxygenation for 2009 Influenza A(H1N1) Acute Respiratory Distress Syndrome. JAMA 302, 1888–1895.
- Gattinoni, L., Pesenti, A., Mascheroni, D., Marcolin, R., Fumagalli, R., Rossi, F., Iapichino, G., Romagnoli, G., Uziel, L., and Agostoni, A. (1986). Low-frequency positive-pressure ventilation with extracorporeal CO2 removal in severe acute respiratory failure. JAMA 256, 881–886.
- Goligher, E.C., Tomlinson, G., Hajage, D., Wijeysundera, D.N., Fan, E., Jüni, P., Brodie, D., Slutsky, A.S., and Combes, A. (2018). Extracorporeal Membrane Oxygenation for Severe Acute Respiratory Distress Syndrome and Posterior Probability of Mortality Benefit in a Post Hoc Bayesian Analysis of a Randomized Clinical Trial. JAMA 320, 2251–2259.
- Guérin, C., Reignier, J., Richard, J.-C., Beuret, P., Gacouin, A., Boulain, T., Mercier, E., Badet, M., Mercat, A., Baudin, O., et al. (2013). Prone positioning in severe acute respiratory distress syndrome. N. Engl. J. Med. 368, 2159–2168.
- Morris, A.H., Wallace, C.J., Menlove, R.L., Clemmer, T.P., Orme, J.F., Jr, Weaver, L.K., Dean, N.C., Thomas, F., East, T.D., Pace, N.L., et al. (1994). Randomized clinical trial of pressure-controlled inverse ratio ventilation and extracorporeal CO2 removal for adult respiratory distress syndrome. Am. J. Respir. Crit. Care Med. 149, 295–305.
- Peek, G.J., Mugford, M., Tiruvoipati, R., Wilson, A., Allen, E., Thalanany, M.M., Hibbert, C.L., Truesdale, A., Clemens, F., Cooper, N., et al. (2009). Efficacy and economic assessment of conventional ventilatory support versus extracorporeal membrane oxygenation for severe adult respiratory failure (CESAR): a multicentre randomised controlled trial. Lancet 374, 1351–1363.
- Pham, T., Combes, A., Rozé, H., Chevret, S., Mercat, A., Roch, A., Mourvillier, B., Ara-Somohano, C., Bastien, O., Zogheib, E., et al. (2013). Extracorporeal membrane oxygenation for pandemic influenza A(H1N1)-induced acute respiratory distress syndrome: a cohort study and propensity-matched analysis. Am. J. Respir. Crit. Care Med. 187, 276–285.
- Richard, J.C., Marque, S., Gros, A., Muller, M., Prat, G., Beduneau, G., Quenot, J.P., Dellamonica, J., Tapponnier, R., Soum, E., et al. (2019). Feasibility and safety of ultra-low tidal volume ventilation without extracorporeal circulation in moderately severe and severe ARDS patients. Intensive Care Med. 45, 1590–1598.
- Rozencwajg, S., Guihot, A., Franchineau, G., Lescroat, M., Bréchot, N., Hékimian, G., Lebreton, G., Autran, B., Luyt, C.-E., Combes, A., et al. (2019). Ultra-Protective Ventilation Reduces Biotrauma in Patients on Venovenous Extracorporeal Membrane Oxygenation for Severe Acute Respiratory Distress Syndrome. Crit. Care Med. 47, 1505–1512.
- Wu, C., Chen, X., Cai, Y., Xia, J. ’an, Zhou, X., Xu, S., Huang, H., Zhang, L., Zhou, X., Du, C., et al. (2020). Risk Factors Associated With Acute Respiratory Distress Syndrome and Death in Patients With Coronavirus Disease 2019 Pneumonia in Wuhan, China. JAMA Intern. Med.
- Zapol, W.M., Snider, M.T., Hill, J.D., Fallat, R.J., Bartlett, R.H., Edmunds, L.H., Morris, A.H., Peirce, E.C., 2nd, Thomas, A.N., Proctor, H.J., et al. (1979). Extracorporeal membrane oxygenation in severe acute respiratory failure. A randomized prospective study. JAMA 242, 2193–2196.
- Ziehr, D.R., Alladina, J., Petri, C.R., Maley, J.H., Moskowitz, A., Medoff, B.D., Hibbert, K.A., Thompson, B.T., and Hardin, C.C. (2020). Respiratory Pathophysiology of Mechanically Ventilated Patients with COVID-19: A Cohort Study. Am. J. Respir. Crit. Care Med.
- (2011). WHO | Seasonal influenza and influenza A(H1N1).
Learn more about research in the Division of Pulmonary and Critical Care Medicine
View all COVID-19 updates