FLARE: The RECOVERY Trial
The FLARE Four
- Yes. Recently released, preliminary, (not yet peer-reviewed) data from the RECOVERY trial demonstrate that dexamethasone has a mortality benefit in COVID-19 patients requiring oxygen or mechanical ventilation and should likely be used
- Prior studies on steroids in ARDS suggested heterogeneous effects, with benefit for some subgroups and harm for others. In particular, observational data on influenza suggests increased mortality with steroid use in viral pneumonia and ARDSnet RCT data suggests harm in patients greater than 13 days from ARDS onset
- Observational data is subject to potential confounding by indication. RCT data from RECOVERY should allay some concerns about harm from low dose steroid use in COVID-19
- Although significant questions remain in regards to longer-term follow-up, use of concurrent medications in the control arm, and further identification of potential effect modifiers, clinicians should strongly consider the use of dexamethasone in their COVID-19 patients with an oxygen requirement or hypoxemic respiratory failure
Many people are asking...should we be using steroids in COVID-19?
Subscribe to the latest updates from FLARE Advances in Motion
Critical Illness, Steroids and Heterogeneity — The RECOVERY Trial
It has been our usual practice with FLARE to discuss peer-reviewed literature. In a break from that usual practice, we devote the entirety of today's FLARE to a review of steroids in ARDS and a new pre-print: the RECOVERY trial data on dexamethasone for COVID-19. The role of steroids in critical illness is highly controversial. Major critical illness syndromes such as septic shock and ARDS are thought to result, in part, from a pathological immune response. From the very first descriptions of these syndromes (Ashbaugh et al. 1967), immunomodulation therapies, including corticosteroids, have been proposed. Immunomodulation for ARDS was reviewed in a prior FLARE (April 4). Widespread adoption of such strategies has been limited by conflicting trial data. With the available literature of insufficient quality to settle the issue, those who favor more widespread use of steroids and those who do not have been left to argue mostly from their own priors.
Analysis of data from the RECOVERY trial highlights a major reason for conflicting results in prior studies—the inherent heterogeneity of critical illness (Iwashyna et al. 2015). In ARDS, as with other critical illness syndromes, clinical disease results from a complex interplay between initial pathologic insult, host immune response, and ongoing injury. Some features of this cascade may be responsive to immunomodulation, some indifferent to it, and others may even be worsened. The existing literature (including RECOVERY) highlights the degree to which the response to steroids may vary not only by individual, but also within a given individual over time. RECOVERY represents important new data with which to approach the question of who might benefit from steroids and who might not.
Why Use Steroids in ARDS?
ARDS may result from a variety of insults (Ware and Matthay 2000) including bacterial and viral pneumonia, aspiration, and extra-pulmonary infection. Regardless of the inciting factor (Huppert, Matthay, and Ware 2019) pathogen exposure, epithelial and/or endothelial injury results in activation of the innate immune system. Pattern recognition receptors recognize molecular signatures on pathogens or damaged host tissues (Mantovani et al. 2011) resulting in neutrophil activation, proinflammatory cytokine (TNF, IL-1, Il-6 and IL-8) release, macrophage recruitment, tissue factor exposure, and coagulation cascade activation (Frantzeskaki, Armaganidis, and Orfanos 2017). This complex pathophysiology results in increased alveolar epithelial and endothelial permeability, impaired alveolar fluid clearance (Huppert, Matthay, and Ware 2019), surfactant dysfunction, V/Q mismatch, and shunt. After the initial phase, patients may experience resolution of inflammation and restoration of normal alveolar function, or instead experience aberrant wound healing with fibroblast infiltration, deposition of collagen and clinical fibrosis (Bachofen and Weibel 1982; Martin et al. 1995).
While the inflammatory cascade described above is activated in response to infection or injury and plays a key role in host defense, there is evidence suggesting that the innate immune response may itself be a source of injury (see Figure 1). Ongoing activation of pattern recognition receptors by dying pathogens and injured host cells may stimulate inflammation longer than is necessary for pathogen removal (Müller-Redetzky et al. 2015). Cyclooxygenase-generated arachidonic acid metabolites elaborated in response to activation of pattern recognition receptors can directly injure epithelial and endothelial cells and result in changes in lung mechanics in animal models of lung injury (Brigham and Meyrick 1986). Neutrophils are common in the bronchoalveolar lavage fluid of ARDS patients and some forms of experimental acute lung injury are neutrophil dependent (Ware and Matthay 2000). There has therefore been a longstanding interest in immunomodulatory strategies to dampen the innate response in ARDS (see Figure 2).
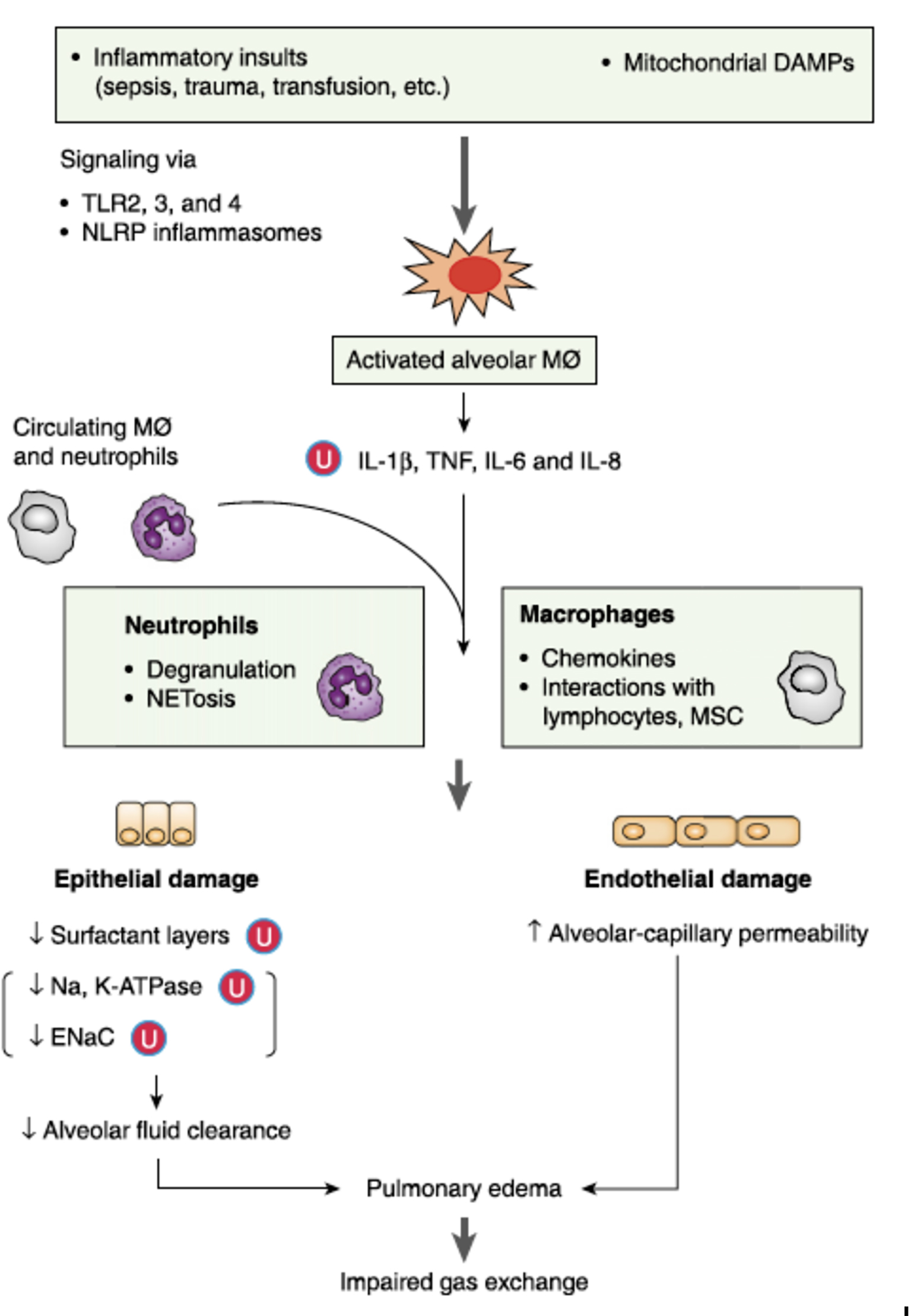
Figure 1
Innate immune system activation and potentially dysregulated immunity as a mechanism of ARDS (Han and Mallampalli 2015).
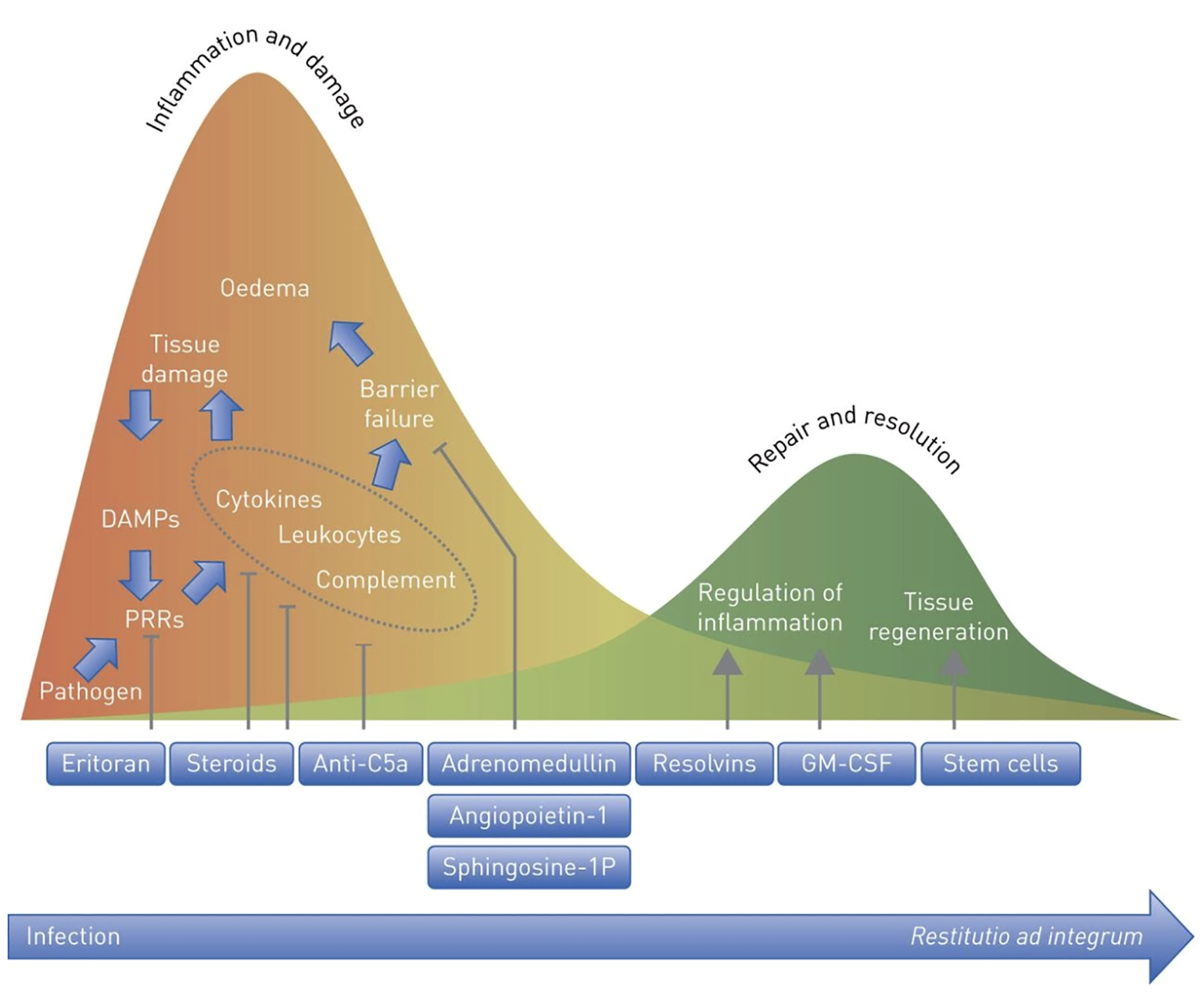
Figure 2
Rationale for immune modulation in lung injury. Appropriate innate immune activation is hypothesized to give way to potentially maladaptive hyperinflammation as disease progresses (Müller-Redetzky et al. 2015).
Glucocorticoids have pleiotropic immunomodulatory effects that may counteract the exuberant inflammation associated with ARDS. Binding of the glucocorticoid receptor by cortisol (Rhen and Cidlowski 2005) exerts anti-inflammatory effects via a number of mechanisms: direct transcriptional regulation via translocation to the nucleus and binding of glucocorticoid response elements by the ligand bound receptor, indirect transcriptional regulation via interactions with other transcriptional regulators such as NF-kB, and non-genomic effects such as liberation of chaperones and other proteins which bind the glucocorticoid receptors in its non-ligand bound form (Cain and Cidlowski 2017). Together, these actions result in decreased expression of proinflammatory cytokines, decreased vascular permeability via decreased expression of vasodilatory compounds (eicosanoids, prostaglandin and bradykinin), and decreased leukocyte recruitment (Figure 2). Glucocorticoids also impair wound healing processes such as collagen deposition, which may be important to preventing fibrosis in ARDS (Martin et al. 1995).
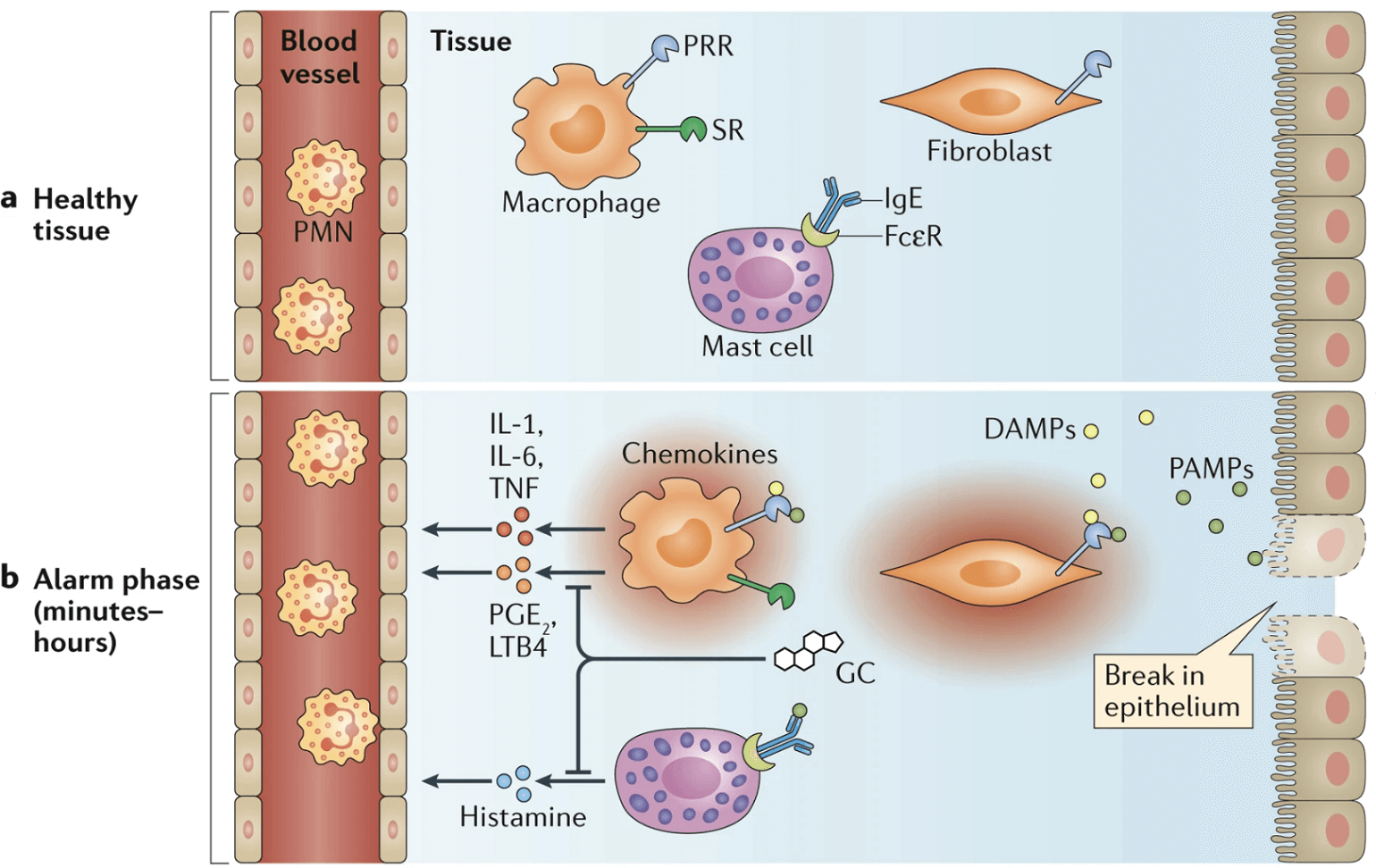
Figure 3
Glucocorticoids decrease expression of pro-inflammatory cytokines (Cain and Cidlowski 2017).
What Prior Trials Have Been Conducted on the Use of Steroids in ARDS?
Prior to RECOVERY, steroids have been extensively studied in ARDS (see Table 1). Before the low tidal volume era, a number of trials were conducted of high-dose, short course corticosteroids with the aim of preventing ARDS. These trials primarily enrolled patients with sepsis rather than ARDS and none showed a benefit with steroid administration. Schein and colleagues (Schein et al. 1987) studied patients with septic shock treated with methylprednisolone, dexamethasone, or standard care and found no difference in rates of ARDS between arms. Weigelt (Weigelt et al. 1986) conducted a single-center RCT of and found a significant increase in severity of respiratory failure and infections in the steroid-treated group. Luce (Luce et al. 1988) and colleagues studied 75 patients with culture-proven sepsis treated with methylprednisolone and found no difference in rate of ARDS between the groups. Finally, Bernard (Bernard et al. 1987) and colleagues studied a similar high dose protocol for established ARDS with a primary endpoint of 45 day mortality and similarly found no difference between the groups.
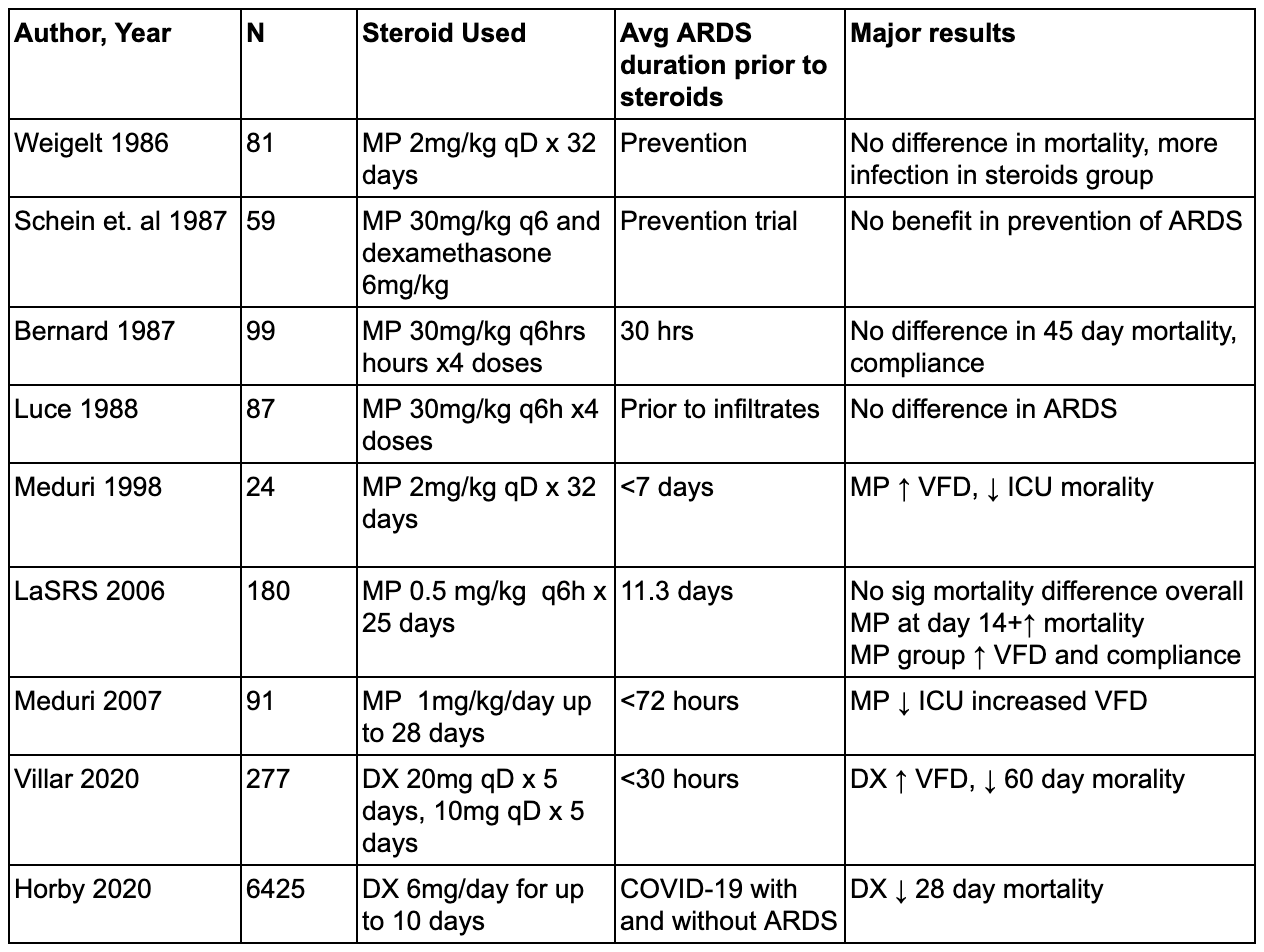
Table 1
Studies of corticosteroid therapy in ARDS. This list is not all-inclusive.
Many of the trials above that did not show a benefit to steroids were conducted prior to significant innovations in supportive care (such as low tidal volume ventilation) and used steroid doses that were significantly higher than what has been tested subsequently. In contrast, a number of investigators have examined lower dose regimens primarily for treatment of established ARDS. Meduri and colleagues (G. U. Meduri et al. 1998) studied 24 patients treated with methylprednisolone at 2mg/kg per day for 32 days. Treatment was initiated, on average, 9 days after diagnosis of ARDS. They found a significant decrease in mortality with steroids. Unfortunately, the study included crossover criteria whereby if a patient failed to improve, they were blindly crossed over to the other treatment group. As a result, nearly half of the placebo group received steroids late in their course, leaving open the possibility that harm from late steroids accounted for part of the difference in the intention-to-treat analysis. Meduri also conducted a somewhat larger trial in 2007 (G. Umberto Meduri et al. 2007) which demonstrated a benefit in terms of markers of inflammation, ICU length of stay and vent free days with early methylprednisolone.
Given the small size of prior trials, the ARDSNet trial group conducted a large, randomized, controlled trial (Steinberg et al. 2006) in which patients with ARDS of greater than 7 days duration were randomized to methylprednisolone. While the primary outcome (60 day mortality) did not differ between treatment and control groups, there were more ventilator-free days in the steroid group. Unfortunately, steroid-treated patients also exhibited increased mortality in the pre-specified subgroup of ARDS > 13 days duration as well as overall greater likelihood of recurrent mechanical ventilation. These harms (postulated but not proven to be related to myopathy) and the crossover effects discussed above together raised concerns that there was an identifiable subgroup (late ARDS) that is harmed by steroid treatment.
More recently, Villar and colleagues studied 277 patients with moderate to severe ARDS across 17 centers in Spain (Villar et al. 2020). The primary endpoint was ventilator-free days up to day 28 and a secondary outcome was 60 day mortality. Inclusion criteria required patients to be enrolled within 30 hours of ARDS onset. The treatment group received 20mg of dexamethasone daily for 5 days, followed by 10mg/day for 5 days. The authors found a significant reduction in 60 day mortality (21% in the treatment group versus 36% in the placebo group). Ventilator-free days and ICU mortality also favored the treatment group, with a similar number of adverse events in both groups. The impressive results of this trial may have been due to early enrollment and selection of a sicker ARDS cohort, but have been criticized for the extremely low rate of enrollment (~3 pts/year per center) and open label design, which raised concerns for selection bias resulting in a treatment group enriched in patients more likely to benefit from steroids.
What About Steroids Specifically for ARDS in the Setting of Viral Pneumonia?
Prior to COVID-19, steroids for viral pneumonia were best studied in influenza, including severe H1N1 influenza. A meta-analysis (Zhang et al. 2015) of 9 studies demonstrated a significant increase in mortality with use of corticosteroids. In addition, a large observational cohort (208 patients) of patients with H1N1 influenza pneumonia and ARDS in France (Brun-Buisson et al. 2011) and a propensity-matched study of 1846 influenza patients in Spain (Moreno et al. 2018) also reported increased mortality with steroid use. Despite this consistent signal for harm, all of this data was observational and potentially suffers from confounding by indication (see April 30th FLARE).
So What Does the Pre-RECOVERY Literature Say as a Whole?
Early negative trials are difficult to interpret given evolving standards of care and high doses of steroids that may have caused harm. A small RCT showed a mortality benefit but with some methodological issues, and two large RCTs studying different steroid regimens applied at different times have conflicting results. In addition, there is a signal for harm in late ARDS (>14 days) and in ARDS due to viral pneumonia. With the exception of viral pneumonia and late ARDS, however, there have been no consistent signals for increased mortality or increased infections with steroids. In addition to the ARDS data, there has been no signal for harm in multiple large randomized trials of steroids for septic shock (Venkatesh et al. 2018; Annane et al. 2018).
One could argue that since steroids appear safe outside of certain defined situations, even a possibility of benefit should favor their use. However, the lack of overall signal for harm in an RCT does not preclude harm to an individual patient who is part of a heterogenous group such as an ARDS cohort (Iwashyna et al. 2015). In addition, since ARDS mortality is typically less than 50%, most patients who meet the Berlin criteria (Bellani et al. 2016) will survive and, among the survivors, steroids have been associated with common post-ICU complications including delirium and neuromuscular weakness (see May 20th FLARE on post-ICU syndrome). Survivors of ARDS experience profound and long lasting muscle weakness (Needham et al. 2014; Herridge et al. 2003) and, in a prospective observational cohort, myopathy and weakness were associated with steroid use (De Jonghe et al. 2002). However, this finding has not been replicated in the large trials detailed above, nor was an increased incidence of weakness seen in two large trials of steroids for septic shock (Venkatesh et al. 2018; Annane et al. 2018). ICU delirium is another significant contributor to post-ICU syndrome and, in another large cohort study, older age and corticosteroid use were independently associated with delirium (Schreiber et al. 2014). In sum, widespread adoption of steroids for ARDS could plausibly result in worsening post-ICU outcomes. This reality must be weighed against any potential benefit.
Okay, Now What About RECOVERY?
For the critical care community, the above discussion (and ongoing debate about steroids) is the context in which the results from the RECOVERY (Horby et al. 2020) trial were recently announced. Currently available only in pre-print form, the RECOVERY trial is a multi-arm, platform trial testing a number of therapies for COVID-19 in parallel and assessing 28 day mortality as a primary endpoint. The sample size is massive: 2104 patients in the steroid arm received 6 mg of dexamethasone daily for up to 10 days and were compared to 4321 patients who received usual care. The authors reported a 3% absolute risk reduction in favor of the steroid group and identified interactions with respiratory support modality, with the greatest benefit seen in those on mechanical ventilation. There was a nonsignificant trend towards harm in patients not requiring oxygen therapy who received steroids (see Figure 4b).
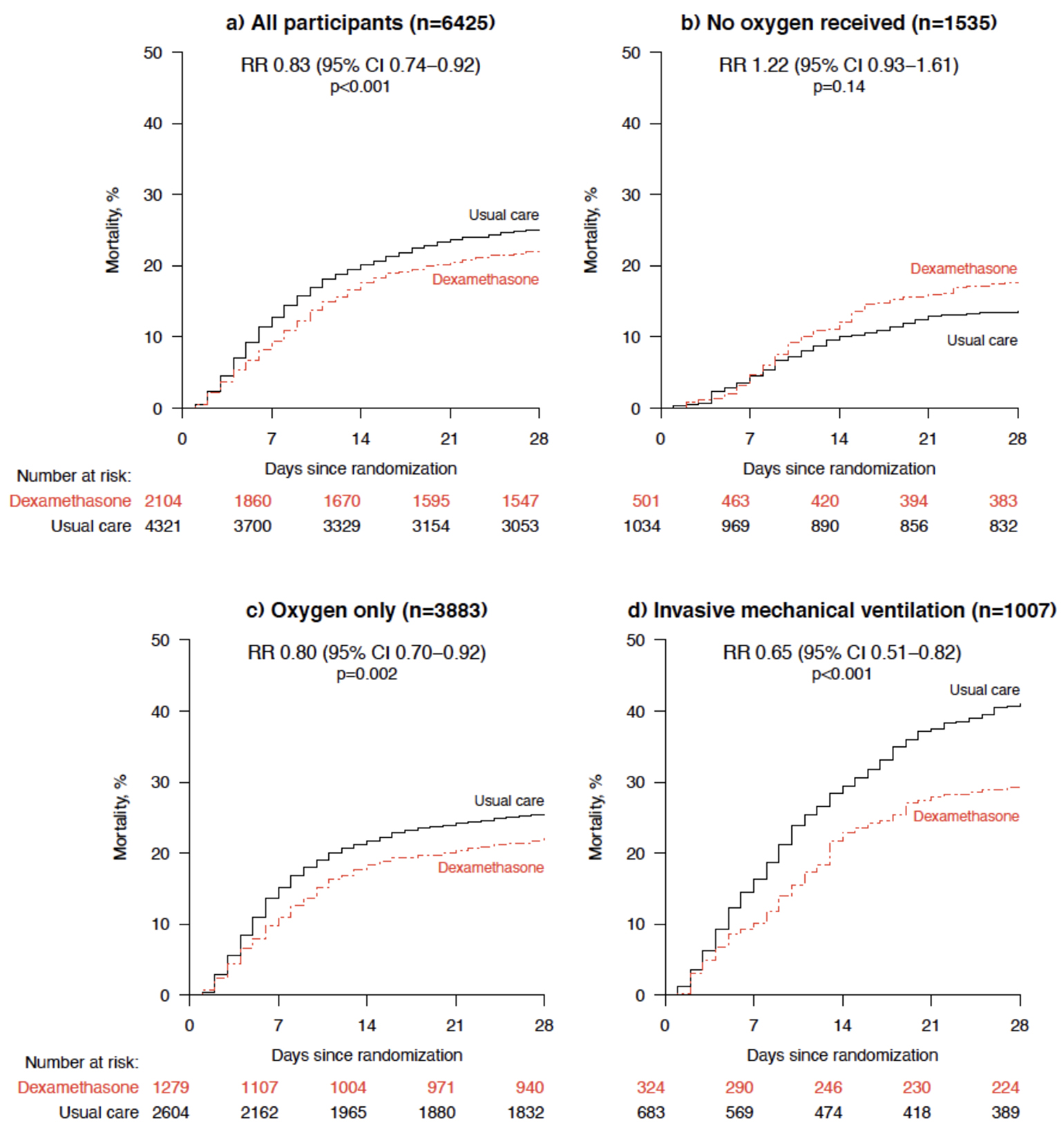
Figure 4
Interaction of between dexamethasone and degree of respiratory support in RECOVERY (Horby et al. 2020).
This interaction between the dexamethasone-associated mortality benefit and the method of oxygen support is interesting, with several possible explanations:
- There is an undefined pathophysiological link between dexamethasone and the method of oxygen support. In other words, the effectiveness of dexamethasone is somehow altered by the provision of supplemental oxygen or mechanical ventilation. However, this seems physiologically implausible.
- Method of oxygen support is a proxy for severity of illness, and the net effect of steroids is a benefit in sicker patients with no effect (or harm) in less sick patients. Notably, the authors included an estimate of likely survival on enrollment (a measure of disease severity) and found no interaction with the benefit of steroids. However, few would argue that someone who is mechanically ventilated is not more sick than someone breathing ambient air, and thus the severity of illness may, in fact, modify the effect of steroids.
- Method of oxygen support is a proxy for the true effect modifier, associated both with the degree of oxygen support and the effect of dexamethasone. For example:
- Method of oxygen support could be a proxy for duration of illness, since COVID-19 patients develop hypoxemia to the point of needing oxygen or ventilation over time. Duration of illness is a biologically plausible effect modifier, since those later in the illness may have less active viral replication and may be more likely to be in a phase of maladaptive immune response. In fact, in another subgroup analysis in the RECOVERY trial, only patients with >7 days since symptom onset had benefit from dexamethasone (Figure S2 in the Preprint Supplement). On the other hand, it has also been reported that severe and prolonged illness is associated with prolonged viral replication (see April 11 FLARE).
- It is also notable that the patients in the mechanical ventilation group (which drives much of the overall benefit observed) are on average 8-10 years younger than the remainder of the cohort (59 years in the “Invasive Mechanical Ventilation” group vs. 67 years in the “Oxygen Only” group vs. 69 years in the “No Oxygen” group). Given this imbalance between groups, method of oxygen support could therefore be a proxy for age, which may be the true effect modifier. This is plausible, since younger patients may either be more likely to benefit from steroids or less likely to be harmed by them. Prior data have not examined steroid effect by age - this would be the first finding of an age-related differential effect.
Elucidating the basis for differential effects by method of oxygen support would have major implications for the application of these trial data. To wit, based on the results presented in the pre-print, dexamethasone should be provided to patients on oxygen or mechanical ventilation. However, if dexamethasone treatment actually interacts with a different variable such as age, duration of symptoms, need for mechanical ventilation, or severity of illness, there is no assurance that the distribution of these variables would be the same outside of the context of the trial.
As the data from RECOVERY are currently limited to a pre-print, there remain other important questions left yet unanswered. The mortality in the untreated patients on mechanical ventilation (40%) is at the higher end of the reported range of mortality in COVID-19 cohorts, with a lower end of the range in the high teens (Ziehr et al. 2020). This mortality rate does not invalidate the benefit of steroids, but raises the possibility of confounders if the control group differed from reference populations in important ways for which we don't yet have data. For example, in this open label trial, concomitant medications may have been differentially given to the control ventilated patients whose providers knew they were not getting steroids. The authors state only that patients did not receive significant treatment with medications being tested in other arms of the trial. However, if other, potentially harmful medications were given more often in the control group this could lead to confounding. This potential confounder is made more plausible by the relatively high control group mortality.
The high mortality observed in the trial also means that the number needed to treat is likely higher at other centers. Again, this does not invalidate the results but, given the potential risk of long term sequelae in survivors treated with steroids, it is worth noting. The current preprint reports outcomes at 28 days; it therefore remains possible that late harm associated with steroids would manifest at 60 or 90 day follow-up. Lastly, the trial is open label, which means that other aspects of care could have been unconsciously influenced by knowledge of the trial intervention, including anticipation of complications of critical illness, increased attention to the patient receiving dexamethasone given predictable effect of steroids such as hyperglycemia, and decisions around duration of life support.
Overall, the data do seem generalizable. However, the large number of patients who died without being intubated, the relatively high mortality in the control group (as mentioned above), and the ability of attending physicians to opt their patients out of the trial if they felt they were likely to be harmed by steroids may all impact the ability to extrapolate the data to another healthcare setting or patient cohort.
Should I Use Steroids for ARDS Now?
The RECOVERY trial enrolled only patients with COVID-19 with and without ARDS and thus, on its face, does not necessarily inform the utility of dexamethasone treatment for ARDS of other etiologies. However, COVID-19 ARDS does not differ meaningfully, either clinically (see April 10th FLARE) or histologically (see May 1st FLARE), from ARDS due to other causes.
Some conclusions may be drawn if the results of RECOVERY are substantiated upon full peer-review and publication:
- First, given the limitations of its open label design, definitive resolution of the question of steroids in ARDS will have to await a truly blinded RCT. The COVID-19 pandemic tragically offers an ongoing and unprecedented opportunity to rapidly enroll ARDS patients in just such a trial.
- Second, the observational data on harm associated with steroid use in viral pneumonia might now be regarded as more likely to be confounded and probably should not guide practice.
- Third, these data, the LaSRS results, and a prior meta-analysis all suggest a modest benefit to steroids in ARDS early but not late (Zhao et al. 2019). The focus in ARDS patients should be within 14 days from ARDS onset.
With the release of these preliminary data, the balance of evidence is now even more in favor of the use of steroids in ARDS; we eagerly await further follow up data to identify any longer term consequences (or benefit).
Conclusions
The use of steroids in critical illness generally, and ARDS in particular, has a long and contentious history. Prior literature consists of older data with unclear applicability to current practice, small trials with significant methodological issues, and large RCTs with conflicting results. Of particular relevance to COVID-19, observational data prior to the current pandemic suggested potential for harm from steroid use in viral pneumonia. The availability (though not yet the publication) of preliminary data from the RECOVERY trial of dexamethasone use in COVID-19 should be practice changing. However, important questions remain. Given concerns for both short and long term adverse events in some patients with steroid use, there remains a need for more precise phenotyping. We must remain alert to the possibility that the groups who appear to benefit in RECOVERY (those on oxygen or mechanical ventilation) are not the true subtypes that distinguish steroid responders from non-responders. In addition, data from open-label trials must continue to be regarded as potentially confounded. Even as we move to a new standard of care of providing dexamethasone to patients with COVID-19, we should continue to call for a fully blinded RCT.
References
- Annane, Djillali, Alain Renault, Christian Brun-Buisson, Bruno Megarbane, Jean-Pierre Quenot, Shidasp Siami, Alain Cariou, et al. 2018. “Hydrocortisone plus Fludrocortisone for Adults with Septic Shock.” The New England Journal of Medicine 378 (9): 809–18.
- Ashbaugh, Davidg, D. Boyd Bigelow, Thomasl Petty, and Bernarde Levine. 1967. “ACUTE RESPIRATORY DISTRESS IN ADULTS.” The Lancet 290 (7511): 319–23.
- Auld, Sara C., Mark Caridi-Scheible, James M. Blum, Chad Robichaux, Colleen Kraft, Jesse T. Jacob, Craig S. Jabaley, et al. 2020. “ICU and Ventilator Mortality Among Critically Ill Adults With Coronavirus Disease 2019.” Critical Care Medicine, May. https://doi.org/10.1097/CCM.0000000000004457.
- Bachofen, M., and E. R. Weibel. 1982. “Structural Alterations of Lung Parenchyma in the Adult Respiratory Distress Syndrome.” Clinics in Chest Medicine 3 (1): 35–56.
- Bellani, Giacomo, John G. Laffey, Tài Pham, Eddy Fan, Laurent Brochard, Andres Esteban, Luciano Gattinoni, et al. 2016. “Epidemiology, Patterns of Care, and Mortality for Patients With Acute Respiratory Distress Syndrome in Intensive Care Units in 50 Countries.” JAMA: The Journal of the American Medical Association 315 (8): 788–800.
- Bernard, Gordon R., John M. Luce, Charles L. Sprung, Jean E. Rinaldo, Robert M. Tate, William J. Sibbald, Khalil Kariman, et al. 1987. “High-Dose Corticosteroids in Patients with the Adult Respiratory Distress Syndrome.” New England Journal of Medicine. https://doi.org/10.1056/nejm198712173172504.
- Bhatraju, Pavan K., Bijan J. Ghassemieh, Michelle Nichols, Richard Kim, Keith R. Jerome, Arun K. Nalla, Alexander L. Greninger, et al. 2020. “Covid-19 in Critically Ill Patients in the Seattle Region - Case Series.” The New England Journal of Medicine 382 (21): 2012–22.
- Brigham, K. L., and B. Meyrick. 1986. “Endotoxin and Lung Injury.” The American Review of Respiratory Disease 133 (5): 913–27.
- Brun-Buisson, Christian, Jean-Christophe M. Richard, Alain Mercat, Anne C. M. Thiébaut, Laurent Brochard, and REVA-SRLF A/H1N1v 2009 Registry Group. 2011. “Early Corticosteroids in Severe Influenza A/H1N1 Pneumonia and Acute Respiratory Distress Syndrome.” American Journal of Respiratory and Critical Care Medicine 183 (9): 1200–1206.
- Cain, Derek W., and John A. Cidlowski. 2017. “Immune Regulation by Glucocorticoids.” Nature Reviews. Immunology 17 (4): 233–47.
- Cummings, Matthew J., Matthew R. Baldwin, Darryl Abrams, Samuel D. Jacobson, Benjamin J. Meyer, Elizabeth M. Balough, Justin G. Aaron, et al. 2020. “Epidemiology, Clinical Course, and Outcomes of Critically Ill Adults with COVID-19 in New York City: A Prospective Cohort Study.” The Lancet, May. https://doi.org/10.1016/S0140-6736(20)31189-2.
- De Jonghe, Bernard, Tarek Sharshar, Jean-Pascal Lefaucheur, François-Jérome Authier, Isabelle Durand-Zaleski, Mohamed Boussarsar, Charles Cerf, et al. 2002. “Paresis Acquired in the Intensive Care Unit: A Prospective Multicenter Study.” JAMA: The Journal of the American Medical Association 288 (22): 2859–67.
- Frantzeskaki, Frantzeska, Apostolos Armaganidis, and Stylianos E. Orfanos. 2017. “Immunothrombosis in Acute Respiratory Distress Syndrome: Cross Talks between Inflammation and Coagulation.” Respiration; International Review of Thoracic Diseases 93 (3): 212–25.
- Han, Seunghye, and Rama K. Mallampalli. 2015. “The Acute Respiratory Distress Syndrome: From Mechanism to Translation.” Journal of Immunology 194 (3): 855–60.
- Herridge, Margaret S., Angela M. Cheung, Catherine M. Tansey, Andrea Matte-Martyn, Natalia Diaz-Granados, Fatma Al-Saidi, Andrew B. Cooper, et al. 2003. “One-Year Outcomes in Survivors of the Acute Respiratory Distress Syndrome.” The New England Journal of Medicine 348 (8): 683–93.
- Horby, Peter, Wei Shen Lim, Jonathan Emberson, Marion Mafham, Jennifer Bell, Louise Linsell, Natalie Staplin, et al. 2020. “Effect of Dexamethasone in Hospitalized Patients with COVID-19: Preliminary Report.” Infectious Diseases (except HIV/AIDS). medRxiv. https://doi.org/10.1101/2020.06.22.20137273.
- Huppert, Laura A., Michael A. Matthay, and Lorraine B. Ware. 2019. “Pathogenesis of Acute Respiratory Distress Syndrome.” Seminars in Respiratory and Critical Care Medicine 40 (1): 31–39.
- Iwashyna, Theodore J., James F. Burke, Jeremy B. Sussman, Hallie C. Prescott, Rodney A. Hayward, and Derek C. Angus. 2015. “Implications of Heterogeneity of Treatment Effect for Reporting and Analysis of Randomized Trials in Critical Care.” American Journal of Respiratory and Critical Care Medicine 192 (9): 1045–51.
- Luce, J. M., A. B. Montgomery, J. D. Marks, J. Turner, C. A. Metz, and J. F. Murray. 1988. “Ineffectiveness of High-Dose Methylprednisolone in Preventing Parenchymal Lung Injury and Improving Mortality in Patients with Septic Shock.” The American Review of Respiratory Disease 138 (1): 62–68.
- Mantovani, Alberto, Marco A. Cassatella, Claudio Costantini, and Sébastien Jaillon. 2011. “Neutrophils in the Activation and Regulation of Innate and Adaptive Immunity.” Nature Reviews. Immunology 11 (8): 519–31.
- Martin, Claude, Laurent Papazian, Marie-Josée Payan, Pierre Saux, and François Gouin. 1995. “Pulmonary Fibrosis Correlates With Outcome in Adult Respiratory Distress Syndrome.” Chest. https://doi.org/10.1378/chest.107.1.196.
- Meduri, G. U., A. S. Headley, E. Golden, S. J. Carson, R. A. Umberger, T. Kelso, and E. A. Tolley. 1998. “Effect of Prolonged Methylprednisolone Therapy in Unresolving Acute Respiratory Distress Syndrome: A Randomized Controlled Trial.” JAMA: The Journal of the American Medical Association 280 (2): 159–65.
- Meduri, G. Umberto, Emmel Golden, Amado X. Freire, Edwin Taylor, Muhammad Zaman, Stephanie J. Carson, Mary Gibson, and Reba Umberger. 2007. “Methylprednisolone Infusion in Early Severe ARDS: Results of a Randomized Controlled Trial.” Chest 131 (4): 954–63.
- Mitra, Anish R., Nicholas A. Fergusson, Elisa Lloyd-Smith, Andrew Wormsbecker, Denise Foster, Andrei Karpov, Sarah Crowe, et al. 2020. “Baseline Characteristics and Outcomes of Patients with COVID-19 Admitted to Intensive Care Units in Vancouver, Canada: A Case Series.” CMAJ: Canadian Medical Association Journal = Journal de l’Association Medicale Canadienne, May. https://doi.org/10.1503/cmaj.200794.
- Moreno, Gerard, Alejandro Rodríguez, Luis F. Reyes, Josep Gomez, Jordi Sole-Violan, Emili Díaz, María Bodí, et al. 2018. “Corticosteroid Treatment in Critically Ill Patients with Severe Influenza Pneumonia: A Propensity Score Matching Study.” Intensive Care Medicine 44 (9): 1470–82.
- Müller-Redetzky, Holger, Jasmin Lienau, Norbert Suttorp, and Martin Witzenrath. 2015. “Therapeutic Strategies in Pneumonia: Going beyond Antibiotics.” European Respiratory Review: An Official Journal of the European Respiratory Society 24 (137): 516–24.
- Needham, Dale M., Amy W. Wozniak, Catherine L. Hough, Peter E. Morris, Victor D. Dinglas, James C. Jackson, Pedro A. Mendez-Tellez, et al. 2014. “Risk Factors for Physical Impairment after Acute Lung Injury in a National, Multicenter Study.” American Journal of Respiratory and Critical Care Medicine 189 (10): 1214–24.
- Rhen, Turk, and John A. Cidlowski. 2005. “Antiinflammatory Action of Glucocorticoids--New Mechanisms for Old Drugs.” The New England Journal of Medicine 353 (16): 1711–23.
- Schein, R. M., R. Bergman, E. H. Marcial, D. Schultz, R. C. Duncan, P. I. Arnold, and C. L. Sprung. 1987. “Complement Activation and Corticosteroid Therapy in the Development of the Adult Respiratory Distress Syndrome.” Chest 91 (6): 850–54.
- Schenck, Edward J., Katherine Hoffman, Parag Goyal, Justin Choi, Lisa Torres, Kapil Rajwani, Christopher W. Tam, Natalia Ivascu, Fernando J. Martinez, and David A. Berlin. 2020. “Respiratory Mechanics and Gas Exchange in COVID-19 Associated Respiratory Failure.” Annals of the American Thoracic Society, May. https://doi.org/10.1513/AnnalsATS.202005-427RL.
- Schreiber, Matthew P., Elizabeth Colantuoni, Oscar J. Bienvenu, Karin J. Neufeld, Kuan-Fu Chen, Carl Shanholtz, Pedro A. Mendez-Tellez, and Dale M. Needham. 2014. “Corticosteroids and Transition to Delirium in Patients with Acute Lung Injury.” Critical Care Medicine 42 (6): 1480–86.
- Steinberg, Kenneth P., Leonard D. Hudson, Richard B. Goodman, Catherine Lee Hough, Paul N. Lanken, Robert Hyzy, B. Taylor Thompson, Marek Ancukiewicz, and National Heart, Lung, and Blood Institute Acute Respiratory Distress Syndrome (ARDS) Clinical Trials Network. 2006. “Efficacy and Safety of Corticosteroids for Persistent Acute Respiratory Distress Syndrome.” The New England Journal of Medicine 354 (16): 1671–84.
- Venkatesh, Balasubramanian, Simon Finfer, Jeremy Cohen, Dorrilyn Rajbhandari, Yaseen Arabi, Rinaldo Bellomo, Laurent Billot, et al. 2018. “Adjunctive Glucocorticoid Therapy in Patients with Septic Shock.” The New England Journal of Medicine 378 (9): 797–808.
- Villar, Jesús, Carlos Ferrando, Domingo Martínez, Alfonso Ambrós, Tomás Muñoz, Juan A. Soler, Gerardo Aguilar, et al. 2020. “Dexamethasone Treatment for the Acute Respiratory Distress Syndrome: A Multicentre, Randomised Controlled Trial.” The Lancet. Respiratory Medicine 8 (3): 267–76.
- Ware, Lorraine B., and Michael A. Matthay. 2000. “The Acute Respiratory Distress Syndrome.” New England Journal of Medicine. https://doi.org/10.1056/nejm200005043421806.
- Weigelt, J. A., J. F. Norcross, K. R. Borman, and W. H. Snyder. 1986. “Early Steroid Therapy for Respiratory Failure.” Survey of Anesthesiology. https://doi.org/10.1097/00132586-198602000-00040.
- Zhang, Yi, Wenjie Sun, Erik R. Svendsen, Song Tang, Raina C. MacIntyre, Peng Yang, Daitao Zhang, and Quanyi Wang. 2015. “Do Corticosteroids Reduce the Mortality of Influenza A (H1N1) Infection? A Meta-Analysis.” Critical Care / the Society of Critical Care Medicine 19 (February): 46.
- Zhao, Qing, Jia-Xin Shi, Rong Hu, Qin Li, Chen-Ying Zhang, and Jia-Shu Li. 2019. “Effect of Glucocorticoids on Mortality in Patients with Acute Respiratory Distress Syndrome: A Meta-Analysis.” Experimental and Therapeutic Medicine 18 (6): 4913–20.
- Ziehr, David R., Jehan Alladina, Camille R. Petri, Jason H. Maley, Ari Moskowitz, Benjamin D. Medoff, Kathryn A. Hibbert, B. Taylor Thompson, and C. Corey Hardin. 2020. “Respiratory Pathophysiology of Mechanically Ventilated Patients with COVID-19: A Cohort Study.” American Journal of Respiratory and Critical Care Medicine, April. https://doi.org/10.1164/rccm.202004-1163LE.
View all COVID-19 Updates
Learn more about research in the Division of Pulmonary and Critical Care Medicine