Additional Therapeutics for SARS-CoV-2
The FLARE Four
- Chloroquine and its potential role in COVID-19
- Data re-analysis and review of recent HCQ +/- Azithromycin trial
- COVID-relative points about ARDS management
- A framework to think about therapeutic approaches
Today's FLARE focus will be on additional therapeutics for SARS-CoV-2.
Subscribe to the latest updates from FLARE Advances in Motion
-
Chloroquine and its potential role in COVID-19
-
Data re-analysis and review of recent HCQ +/- Azithromycin trial
-
COVID-relative points about ARDS management
-
Use of methylprednisolone in ARDS via the LaSRS Trial
-
ARDS phenotyping and differential response to treatments
-
Below is a framework to think about therapeutic approaches followed by data about specific therapies:
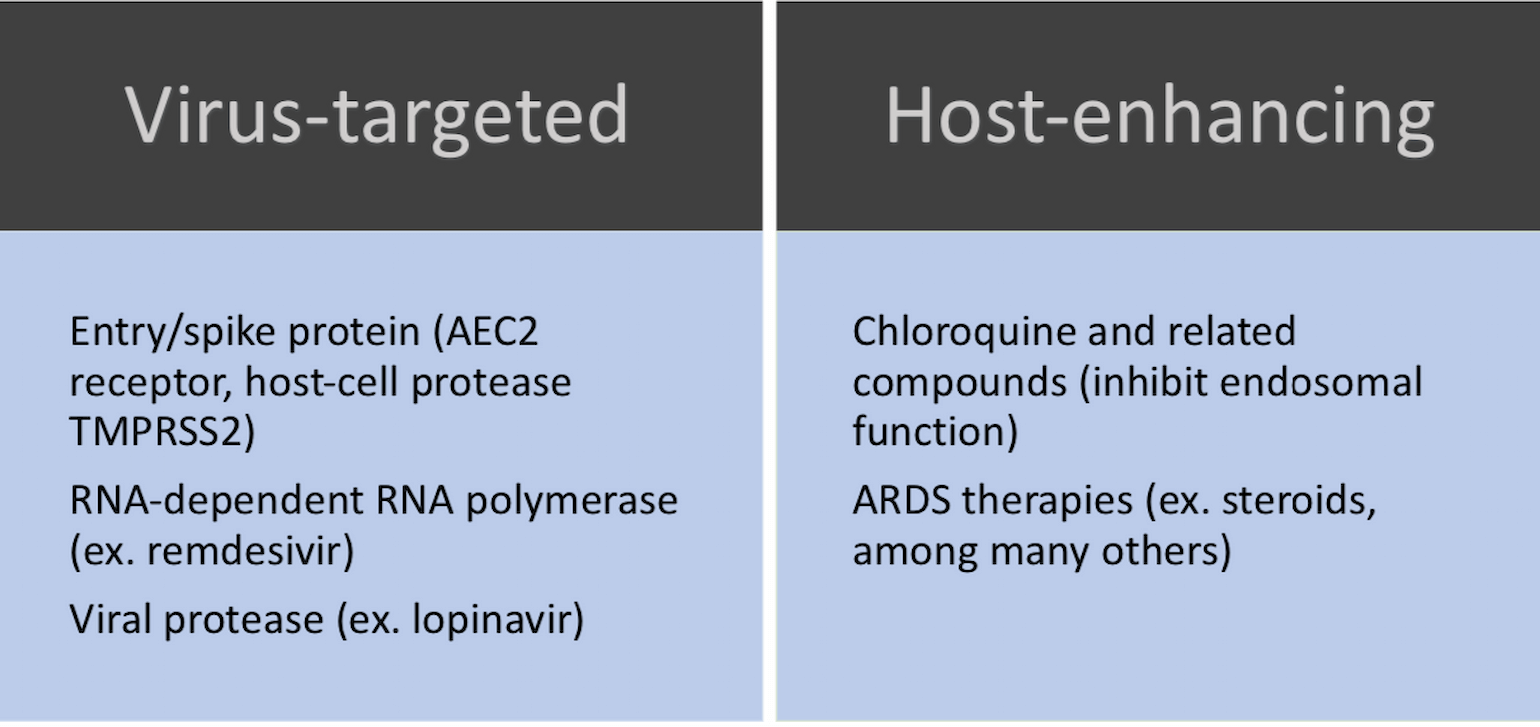
Figure 1
Chloroquine: A Q&A with Dr. Raghu Chivukula
What is the Deal with Chloroquine?
Let’s start with a review of some virology basics. Coronaviruses are enveloped RNA viruses, meaning they are coated in a piece of host cell membrane. There are several families of Coronaviruses which differ in the receptors they use for host entry, but several papers in the last decade or so have revealed an important role for endocytosis in viral entry. Through this process the entire virus (envelope and all) is internalized, and the endocytosed virion therefore ends up inside a membrane-bound compartment in the cell (an endosome) from which it must escape before making mischief in the nucleus. This trafficking and escape process generally depends on acidification of the endosome and/or fusion with acidified lysosomes.
OK. So what does this have to do with chloroquine (CQ)? CQ belongs to a class of agents known as “cationic amphiphilic drugs” (CADs) which share some peculiar structural features - most notably an amino group on one end. When these drugs encounter acidified compartments, their amino groups become protonated and the molecules become trapped. The end result is that CADs accumulate to very high concentrations in normally acidified compartments like the endosome, lysosome, and trans-Golgi apparatus and have a variety of effects on the enzymes and macromolecules that reside there. For example, this phenomenon is the mechanistic basis for phospholipidosis (“foamy macrophages”) in patients treated with the cationic amphiphilic drug amiodarone. Each CAD induces unique but overlapping changes in lysosomal and Golgi metabolism in complex ways specific to its chemical composition. It should be noted here that the exact effects of CQ on lysosomes and endosomes remains an area of active research.
Nevertheless, it has been reported for decades that chloroquine, amiodarone, and other CADs can interfere with the replication of a diverse array of enveloped viruses including influenza, Ebola virus, HIV, Dengue, Zika, and HCV in vitro. On the other hand, in vivo assays and clinical trials have been less promising – chloroquine failed to prevent influenza infection in a clinical trial (Paton, et. al. Lancet Inf Dis. 2011) and, if anything, increased viral load in an HIV trial (Paton, et. al. JAMA 2012). And, concerningly, at least one group reported increased influenza replication in the presence of CQ (Wu, et. al. J Med Vir 2015).
Why Do People Think CQ/HCQ Might Be Effective Against SARS-Cov-2?
The majority of evidence supporting the use of CQ and its derivative, hydroxychloroquine (HCQ), come from studies of the original SARS-Cov which emerged in 2002. In the ensuing years, basic virology studies established that SARS-Cov depends on endosomal escape, that it buds from the Golgi apparatus, and that its receptor (ACE2) is itself glycosylated in the Golgi. Thus, CQ was a rational drug to test. Vincent and colleagues established the efficacy of CQ in inhibiting SARS-Cov replication and provided evidence that impaired endosomal acidification as well as impaired ACE2 glycosylation might be responsible. Similar data were reported by other groups (Keyrts, et. al. Biochem Biophys Res Commun. 2004).
Based on these data, Wang and colleagues published two papers in Feb (Wang, et. al. Cell Research2020) and March (Liu, et. al. Cell Discovery 2020) of this year examining the effects of CQ and HCQ (more widely available and less toxic) on the novel SARS-Cov-2 in vitro. CQ dramatically inhibits SARS-Cov-2 replication at low micromolar concentrations, while HCQ inhibits replication at ~10uM. Importantly, these concentrations are at least 10-fold lower than reported cytotoxic doses – though it is virtually certain normal cellular physiology is being perturbed to some extent.
What about clinical evidence? As of this writing (March 21), there are no rigorous clinical data specifically demonstrating therapeutic benefit of CQ or HCQ in prevention or treatment of COVID-19 save for a “publication” (Gao, et. al. BioScience Trends 2020) which states without evidence that CQ has proven effective in >100 patients. There are, however, >20 active clinical trials (most in China) and it is likely that data will emerge in the coming weeks. Which brings us to ...
"Many People are Saying" That HCQ and Azithromycin Together Cure COVID-19. Is That True?
If you have been following the news, you likely saw that President Trump voiced a great deal of enthusiasm for combination therapy with hydroxychloroquine and azithromycin (“H and A”, as he coined). This drug combination was studied in a very small group of French patients, published a couple of days ago (Gautret, et. al. Intl J Antimicrob Agents 2020). Let’s walk through the paper:
- Study population: SARS-Cov-2 positive adults (asymptomatic and symptomatic) who expressed interest in receiving HCQ. Controls were taken from another center or from patients who refused the drug
- Design: Patients were offered 200mg TID HCQ. Patients had NP swabs taken daily for RT-PCR of viral load. Serum was collected for HCQ measurement. Antibiotic therapy (azithromycin) was provided based on clinical judgement
- Inclusion/exclusion: 42 met inclusion criteria -> 6 patients lost (3 to ICU, 1 died, 1 left hospital, 1 stopped drug) -> 36 patients completed study -> 26 HCQ + 16 control. 6 of the HCQ patients also received azithromycin based on clinical judgement “to prevent bacterial super-infection”
- Results: HCQ serum levels were 1.4±0.6 uM in the 20 patients with data available. Patients treated with HCQ had statistically significantly higher rates of “virological cure” than those not-treated, beginning at day 3 of treatment. The 6 patients treated with HCQ/azithromycin had “100% virological cure” beginning at day 5
There are several significant methodological issues with this study beyond its small size (which is excusable). Most glaring is that qRT-PCR was only performed on half the controls, while the remainder were assayed only as “present” versus “absent”. Second, among those patients who had qRT-PCR performed, no QC or normalization of any kind appeared to be performed – meaning, for example, if a swab did not really obtain cells we would not know. Third, 5 of the 6 HCQ patients not analyzed were omitted because they had clinical deterioration; had they been included, they likely would have been treatment failures. Fourth, the decision to administer azithromycin was entirely ad hoc, making it highly suspect to conclude pharmacological synergy from these data. Finally, my own reanalysis of these data (asking what the maximal observed reduction in signal was per patient) suggests treatment effect is insignificant. Happy to share this analysis with any interested parties – they are very imperfect given the starting material. In conclusion, I don’t find this study compelling but the question needs to be asked in a much more rigorous fashion to know for sure.
What is Known About ARDS That May Apply to COVID-19?
A retrospective cohort study in China recently purported that methylprednisolone may decrease the risk of death in COVID-19-related ARDS. A Chinese hospital also announced a single-center RCT of methylprednisolone vs placebo for adjunctive therapy of COVID-19-related severe lower respiratory tract infection.
A Refresher: What is the Evidence for Corticosteroids in ARDS?
The LaSRS Trial (2006)
Methods: Double-blind RCT of 180 patients with late ARDS (at least 7 days duration) randomized to receive moderate-dose methylprednisolone or placebo. Primary endpoint was mortality at 60 days.
Results: No significant difference was found in mortality at 60 or 180 days between methylprednisolone or placebo groups. HOWEVER, methylprednisolone treatment was associated with significantly INCREASED 60 and 180 day mortality among patients enrolled at least 14 days after onset of ARDS. Methylprednisolone did increase the number of ventilator-free and shock-free days during the first 28 days after enrollment, and this was associated with improved oxygenation and cardiopulmonary physiology during that time.
Conclusion: Results did not support routine methylprednisolone for persistent ARDS, despite early improvement in cardiopulmonary physiology.
Several earlier trials in the 1980s of corticosteroids for early-phase ARDS failed to show improvement in survival.
Thus far, there is no indication that ARDS due to SARS-CoV-2 infection is pathophysiologically different from other causes of ARDS. Therefore, the above findings that steroids are not helpful and potentially harmful in the management of ARDS likely hold true in this population.
Prior work from Dr. Carolyn Calfee's group has demonstrated that ARDS patients may be categorized into two subphenotypes. These two phenotypes are clinically distinct (hyperinflammatory, and hypoinflammatory) and have differential responses to ARDS therapies, some of which are being explored for COVID-19 disease. Below we review these studies.
Famous KR, Delucchi K, Ware LB, et al. Acute Respiratory Distress Syndrome Subphenotypes Respond Differently to Randomized Fluid Management Strategy. AJRCCM 2017;195:331-8.
A previous analysis done by this group identified 2 ARDS sub-phenotypes using data from the ARMA and ALVEOLI trials. Subphenotype 2 was associated with increased inflammation, acidosis and shock. In this analysis, they confirmed their findings using an independent cohort from the FACTT trial (N=1000). They used latent class analysis and biomarker data to identify subphenotypes and confirmed a two-subphenotype model describing the population. This model estimated 727 subjects for class I and 273 in class II. Latent class probabilities were 0.97 for class I and 0.93 for class 2. Subphenotype 2 had higher levels of IL-8, IL-6, Ang-2, RAGE and STNFr1, but lower protein C and bicarbonate. Overall, subphenotype 2 again showed higher inflammatory markers and hypotension. While a greater proportion of subphenotype 2 had sepsis as their ARDS risk factor, subjects with sepsis were equally as likely to be assigned to either subphenotype. Subjects with trauma, aspiration or pneumonia were more likely to be assigned subphenotype 1. Overall, subphenotype 2 had higher 60- and 90-day mortality with fewer ventilator free days than those assigned to subphenotype 1. Logistic regression was used to test for interaction between subphenotype and treatment. Patients assigned to subphenotype 1 had a 90-day mortality of 26% when randomized to fluid-liberal strategy (vs 18% in fluid conservative strategy). Patients in subphenotype 2 had 40% mortality when randomized to fluid liberal strategy vs 50% in fluid conservative strategy. Both subphenotypes were significant effected by fluid management strategy. They were then able to use a stepwise model to generate a model for subphenotype evaluation. A three-variable model of IL-8, bicarbonate and sTNFr1 accurately classify the subphenotypes (AUC ranges from 0.91 to 0.95 in different cohorts).
Take away point: There are subtypes of ARDS that may respond differently to specific treatments.
Calfee CS, Delucchi KL, Sinha P et al. Acute respiratory distress syndrome subphenotypes and differential response to simvastatin: secondary analysis of a randomised controlled trial. Lancet Respir Med. 2018;6(9):691-698.
Calfee et al. again applied the same latent class analysis methods to baseline data from the HARP-2 randomized controlled trial of simvastatin for ARDS treatment and again identified two distinct classes, termed hypoinflammatory (~65% of study participants) and hyperinflammatory (35%), which had similar features to those described in Famous et al.. They found that the hyperinflammatory subphenotype had improved survival with simvastatin compared to placebo.
With all this mind, we wonder about the role of some of the currently suggested therapies. For example, immunomodulatory therapies may be detrimental to patients of the hypoinflammatory subphenotype. We anticipate much more discussion and discovery here in the coming weeks.
References
- Paton NI, Lee L, Xu Y, et al. Chloroquine for influenza prevention: a randomised, double-blind, placebo controlled trial [published correction appears in Lancet Infect Dis. 2011 Sep;11(9):655.
- Paton NI, Goodall RL, Dunn DT, et al. Effects of hydroxychloroquine on immune activation and disease progression among HIV-infected patients not receiving antiretroviral therapy: a randomized controlled trial. JAMA. 2012;308(4):353–361.
- Wu L, Dai J, Zhao X, Chen Y, Wang G, Li K. Chloroquine enhances replication of influenza A virus A/WSN/33 (H1N1) in dose-, time-, and MOI-dependent manners in human lung epithelial cells A549. J Med Virol. 2015;87(7):1096–1103.
- Wang M, Cao R, Zhang L, et al. Remdesivir and chloroquine effectively inhibit the recently emerged novel coronavirus (2019-nCoV) in vitro. Cell Res. 2020;30(3):269–271.
- Liu, J., Cao, R., Xu, M. et al. Hydroxychloroquine, a less toxic derivative of chloroquine, is effective in inhibiting SARS-CoV-2 infection in vitro. Cell Discov 6, 16 (2020).
- Gao J, Tian Z, Yang X. Breakthrough: Chloroquine phosphate has shown apparent efficacy in treatment of COVID-19 associated pneumonia in clinical studies. Biosci Trends. 2020;14(1):72–73.
- Gautret P, Lagier J, Parola P, Hoang V, Meddeb L, Mailhe M, et al. Hydroxychloroquine and azithromycin as a treatment of COVID-19: results of an open-label non-randomized clinical trial. International Journal of Antimicrobial Agents. In Press.
- Steinberg KP, Hudson LD, Goodman RB et al. Efficacy and safety of corticosteroids for persistent acute respiratory distress syndrome. N Engl J Med. 2006; 354: 1671-1684
- Bone RC, Fisher CJ Jr, Clemmer TP, Slotman GJ, Metz CA. Early methylprednisolone treatment for septic syndrome and the adult respiratory distress syndrome. Chest 1987;92:1032-6.
- Luce JM, Montgomery AB, Marks JD, Turner J, Metz CA, Murray JF. Ineffectiveness of high-dose methylprednisolone in preventing parenchymal lung injury and improving mortality in patients with septic shock. Am Rev Respir Dis 1988;138: 62-8.
- Bernard GR, Luce JM, Sprung CL, et al. High-dose corticosteroids in patients with the adult respiratory distress syndrome. N Engl J Med 1987;317:1565-70.
- Weigelt JA, Norcross JF, Borman KR, Snyder WH III. Early steroid therapy for respiratory failure. Arch Surg 1985;120: 536-40.
- Famous KR, Delucchi K, Ware LB, et al. Acute Respiratory Distress Syndrome Subphenotypes Respond Differently to Randomized Fluid Management Strategy. AJRCCM 2017;195:331-8.
- Calfee CS, Delucchi KL, Sinha P et al. Acute respiratory distress syndrome subphenotypes and differential response to simvastatin: secondary analysis of a randomised controlled trial. Lancet Respir Med. 2018;6(9):691-698.
View all COVID-19 updates
Learn about research in the Division of Pulmonary and Critical Care Medicine