Will SARS-CoV-2 Disappear in the Summer?
The FLARE Four
- Respiratory viral infections often have a defined seasonality
- The determinants of seasonality include host behavior, changes in immunity, as well as absolute humidity and temperature
- Pandemics often do not adhere to typical viral seasonality
- We do not know whether transmission of SARS-CoV-2 will be different in the summer, but there is hope that viral transmission could be reduced
Many people are saying... “...There’s a theory that, in April, when it gets warm — historically, that has been able to kill the virus. So we don’t know yet; we’re not sure yet. But that’s around the corner.”
Subscribe to the latest updates from FLARE Advances in Motion
Circulating Viruses Have Seasonality
All diseases occur at all seasons of the year, but certain of them are more apt to occur and be exacerbated at certain seasons.
-Hippocrates Aphorisms, III, 19
The idea that respiratory viral infection has seasonal oscillations goes back to the time of Hippocrates and is so well established that it has entered our common lexicon with the term “flu season.” Indeed, most of the common endemic respiratory viral pathogens have a seasonality with peak incidence in specific seasons. For example, in temperate climates, endemic influenza, coronavirus, and respiratory syncytial virus (RSV) all show peak incidence in the winter months and have been termed “winter viruses,” while non-rhinovirus enterovirus has a peak incidence in the summer months and has been termed a “summer virus.” Other respiratory viruses such as adenovirus, rhinovirus, and human metapneumovirus are detected throughout the year, while some viruses such as parainfluenza have type-specific seasonal circulation (Moriyama, Hugentobler, and Iwasaki 2020). Interestingly, in tropical climates, the seasonality of influenza is less well defined and active virus circulates throughout the year (Viboud, Alonso, and Simonsen 2006).
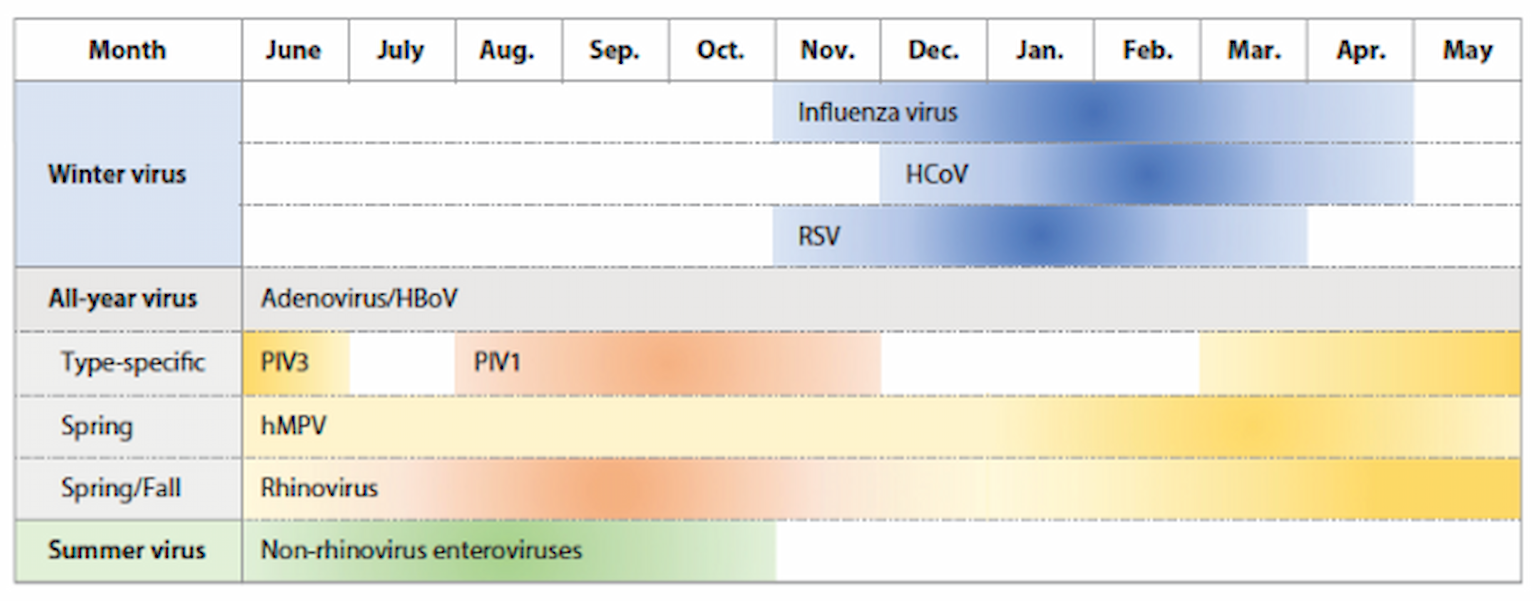
Figure 1
Schematic of seasonality of respiratory virus infection in temperate regions (Moriyama et al. 2020).
Are Coronaviruses Seasonal? Do We Have Reason to Suspect the Same for SARS-CoV-2?
Both SARS and COVID-19 emerged in the winter months. The SARS outbreak began in China’s Guangdong province in November of 2002 and circulated in as many as 29 countries until the spring of 2004. COVID-19 emerged in Wuhan, China in December 2019 and remains widely circulating at this time. A non-peer reviewed report published in MedRxiv (Bannister-Tyrrell et al. 2020) recently provided preliminary data that countries with higher temperatures appeared to have modest reductions in COVID-19 incidence. However, this study is confounded by differences in travel, testing, and containment strategies between different countries. Better evidence comes from a large-scale comprehensive screening program for the endemic circulating coronaviruses (229E, NL63, HKU1, and OC43) in the UK during 2006-2009 (Gaunt et al. 2010). This study demonstrated marked winter seasonality with coronavirus infections predominantly seen between the months of December and April. These observations have led some to propose that summer will reduce the incidence of SARS-CoV-2 infection in the northern hemisphere.
What Were the Results?
“Unfortunately, this potpourri of possible mechanisms places us in a kind of Popperian Purgatory, in which data in support of every hypothesis exist, yet none of the hypotheses has been subjected to tests that are rigorous enough to reject it.”
- Lipsitch and Viboud 2009
There are several hypotheses that may explain seasonal variation in respiratory viral infection, including:
1. Changes in Host Behavior
Changes in human behavior during the winter months have been proposed to influence seasonality. This includes gathering indoors, holiday travel, and aggregation of children in schools. However, as humans in developed countries spend more than 90% of their time indoors throughout the year, seasonal changes in the degree of indoor congregation seems unlikely to have a large effect (Moriyama, Hugentobler, and Iwasaki 2020). In addition, travel in the summer months is also very active and not associated with increased risk. The best data for the association of seasonal variation of human behavior and virus incidence are from the 2009 H1N1 pandemic. In Mexico, Hong Kong and Europe, school closures resulted in a 25-30% reduction in viral transmission (Fisman 2012). Resurgence of another wave of H1N1 influenza in Mexico began 2-5 weeks after the beginning of the fall term (Chowell et al. 2011). This suggests that for influenza, school closures may have significant effects on virus transmission.
2. The Effects of Climate on Host Defense
Muco-ciliary clearance (MCC) is critical for host defense in the lung. Ciliary beating of nasal and tracheal cells is impaired at 20°C and ceases at 5°C in vitro (Clary-Meinesz et al. 1992). Furthermore, dry air can cause mucus dehydration and impaction. These changes are likely to significantly impair MCC in humans and this hypothesis has been experimentally demonstrated in mice and chickens exposed to cold dry air (Kudo et al. 2019; Baetjer 1967). In addition to its effects on MCC, cooler air (33°C versus 37°C) has also been demonstrated to impair innate interferon responses to rhinovirus, allowing for more robust viral replication at cooler temperatures (Foxman et al. 2015). Mice exposed to influenza at lower relative humidity had worsened outcomes and this was associated with a global reduction of interferon-stimulated gene expression in the lung, also suggesting a defect in interferon production in dry cool air (Kudo et al. 2019). Finally, Vitamin D has been proposed to alter innate and adaptive immune responses and increased vitamin D deficiency in the winter has been proposed to contribute to infection as well (Fisman 2012).
3. The Effect of Temperature and Humidity on Virus Survival and Transmission
Guinea pig models have demonstrated that temperature and relative humidity may alter influenza transmission (Lowen et al. 2007). However, the mechanism was not well understood until Shaman and Kohn revisited these studies (Shaman and Kohn 2009). They reinterpreted the results of the guinea pig studies in terms of absolute humidity (or vapor pressure) and found that transmission decreased with decreasing vapor pressure in a linear relationship. They then went on to show that vapor pressure was directly related to the duration of viable virus in aerosols. In addition, it has been proposed that low temperatures promote stability of the influenza virus particle by effects on the viral membrane lipids (Polozov et al. 2008). The role of low temperatures and absolute humidity was then confirmed on a population level showing that the onset of influenza mortality in the U.S. is associated with episodes of low absolute humidity during the previous weeks (Shaman et al. 2009). Thus, low temperature and absolute humidity may strongly influence influenza transmission in temperate climates. Confusingly, very humid weather in the tropics also appears to promote influenza transmission. These conditions have been proposed to be poor for aerosol spread, but may enhance spread by direct contact and fomites (Lowen et al. 2008).
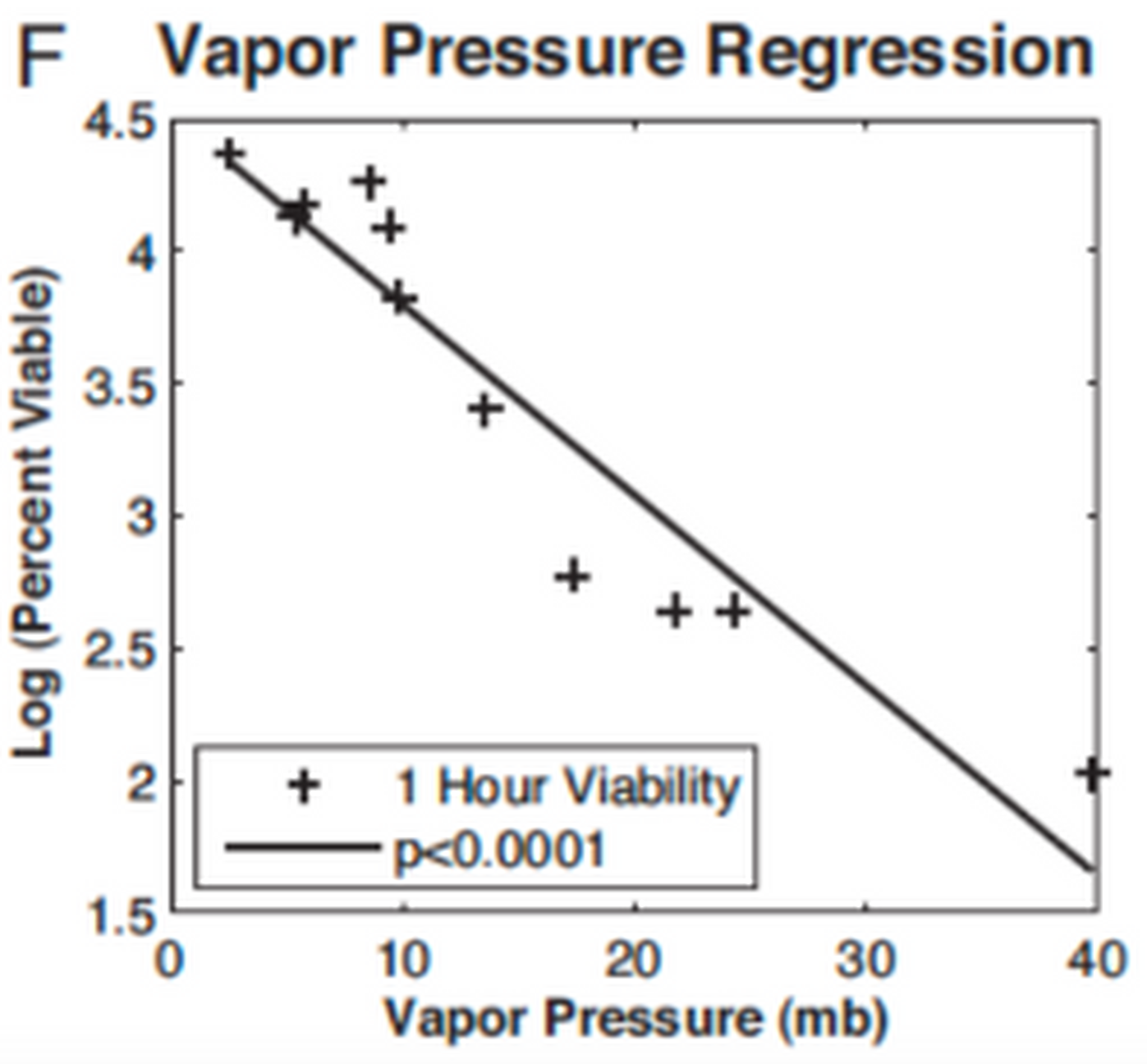
Figure 2
1-hour viability and vapor pressure (Shaman and Kohn 2009).
4. Interaction with Other Viruses
Susceptible infection recovery modeling predicts that cross-reactive immunity between different influenza strains results in seasonal oscillations of these strains (Lofgren et al. 2007). This likely is determined by the level of heterosubtypic immunity (cross protection to infection by one viral serotype after infection by a different serotype) and duration of immunity. In addition, there is a strong negative interaction between influenza, rhinovirus and RSV (Pnestad 1982; Nickbakhsh et al. 2019). This suggests that having one respiratory virus infection may provide some form of cross-protection from another but the immunologic mechanisms underlying this observation are unclear. Finally, even without seasonal variation, as an epidemic progresses and individuals are infected and then become immune, the percentage of the susceptible population declines, leading to a decline in infection rates with an oscillation frequency that is determined by the durability of immunity and influx of susceptible hosts.
Influenza Pandemics Had a Different Seasonality from Epidemic Influenza
Pandemic respiratory viral infection has often not followed the same seasonality of endemic respiratory viruses. In fact, all of the recent influenza pandemics arose in the northern hemisphere in the spring and summer months with the 1918 pandemic emerging in March, the 1957 and 2009 pandemics in April, and the 1968 pandemic in July. For the 1918, 1957 and 2009 pandemics, the initial herald wave was followed by a second or third more geographically dispersed wave the following fall or winter (Fox, Miller, and Meyers 2017). It has been hypothesized that the original and subsequent waves of infection were influenced by interaction with circulating seasonal epidemic influenza, the changes in weather, and the effects in social grouping, particularly the relation to the school year. However, the relative contributions of each of these factors has been very difficult to determine.
Bottom Line: Will SARS-CoV-2 Transmission Be Affected By Seasonal Variation?
At this point, we just don’t know. As shown above, there are a multitude of parameters that affect viral transmission. It should be considered that pandemic viruses appear to have unique circulating properties compared to endemic viruses likely due to a lack of pre-existing immunity. It should also be noted that SARS was not stopped by changes in the weather but was contained by aggressive contact tracing and intensive public health intervention. However, influenza virus transmission and survival are strongly influenced by absolute humidity and temperature as well as on seasonal changes in host activity and immunity. It is possible that like influenza, the mechanism of transmission may change based on the weather with cold, dry air promoting aerosol spread and warm, humid air promoting contact and fomite spread. Thus, we should study if vapor pressure also affects coronavirus stability and determine if indoor humidification would be of benefit in reducing transmission in school and hospital settings. Furthermore, mask wearing may increase the temperature and humidity of inhaled air and thus may provide benefits on MCC and innate interferon responses.
Thus, it is possible that viral transmission may be somewhat affected by the coming summer months in the northern hemisphere, but this hope should not replace social distancing, mask wearing and other containment methods until we understand the dynamics of transmission better.
References
- Baetjer, A. M. 1967. “Effect of Ambient Temperature and Vapor Pressure on Cilia-Mucus Clearance Rate.” Journal of Applied Physiology 23 (4): 498–504.
- Bannister-Tyrrell, Melanie, Anne Meyer, Celine Faverjon, and Angus Cameron. 2020. “Preliminary Evidence That Higher Temperatures Are Associated with Lower Incidence of COVID-19, for Cases Reported Globally up to 29th February 2020.” Epidemiology. medRxiv. https://doi.org/10.1101/2020.03.18.20036731.
- Chowell, Gerardo, Santiago Echevarría-Zuno, Cécile Viboud, Lone Simonsen, James Tamerius, Mark A. Miller, and Víctor H. Borja-Aburto. 2011. “Characterizing the Epidemiology of the 2009 Influenza A/H1N1 Pandemic in Mexico.” PLoS Medicine 8 (5): e1000436.
- Clary-Meinesz, C. F., J. Cosson, P. Huitorel, and B. Blaive. 1992. “Temperature Effect on the Ciliary Beat Frequency of Human Nasal and Tracheal Ciliated Cells.” Biology of the Cell. https://doi.org/10.1016/0248-4900(92)90436-5.
- Fisman, D. 2012. “Seasonality of Viral Infections: Mechanisms and Unknowns.” Clinical Microbiology and Infection: The Official Publication of the European Society of Clinical Microbiology and Infectious Diseases 18 (10): 946–54.
- Foxman, Ellen F., James A. Storer, Megan E. Fitzgerald, Bethany R. Wasik, Lin Hou, Hongyu Zhao, Paul E. Turner, Anna Marie Pyle, and Akiko Iwasaki. 2015. “Temperature-Dependent Innate Defense against the Common Cold Virus Limits Viral Replication at Warm Temperature in Mouse Airway Cells.” Proceedings of the National Academy of Sciences of the United States of America 112 (3): 827–32.
- Fox, Spencer J., Joel C. Miller, and Lauren Ancel Meyers. 2017. “Seasonality in Risk of Pandemic Influenza Emergence.” PLoS Computational Biology 13 (10): e1005749.
- Gaunt, E. R., A. Hardie, E. C. J. Claas, P. Simmonds, and K. E. Templeton. 2010. “Epidemiology and Clinical Presentations of the Four Human Coronaviruses 229E, HKU1, NL63, and OC43 Detected over 3 Years Using a Novel Multiplex Real-Time PCR Method.” Journal of Clinical Microbiology. https://doi.org/10.1128/jcm.00636-10.
- Kudo, Eriko, Eric Song, Laura J. Yockey, Tasfia Rakib, Patrick W. Wong, Robert J. Homer, and Akiko Iwasaki. 2019. “Low Ambient Humidity Impairs Barrier Function and Innate Resistance against Influenza Infection.” Proceedings of the National Academy of Sciences of the United States of America 116 (22): 10905–10.
- Lipsitch, Marc, and Cécile Viboud. 2009. “Influenza Seasonality: Lifting the Fog.” Proceedings of the National Academy of Sciences of the United States of America.
- Lofgren, Eric, N. H. Fefferman, Y. N. Naumov, J. Gorski, and E. N. Naumova. 2007. “Influenza Seasonality: Underlying Causes and Modeling Theories.” Journal of Virology 81 (11): 5429–36.
- Lowen, Anice C., Samira Mubareka, John Steel, and Peter Palese. 2007. “Influenza Virus Transmission Is Dependent on Relative Humidity and Temperature.” PLoS Pathogens 3 (10): 1470–76.
- Lowen, Anice C., John Steel, Samira Mubareka, and Peter Palese. 2008. “High Temperature (30 Degrees C) Blocks Aerosol but Not Contact Transmission of Influenza Virus.” Journal of Virology 82 (11): 5650–52.
- Moriyama, Miyu, Walter J. Hugentobler, and Akiko Iwasaki. 2020. “Seasonality of Respiratory Viral Infections.” Annual Review of Virology, March. https://doi.org/10.1146/annurev-virology-012420-022445.
- Nickbakhsh, Sema, Colette Mair, Louise Matthews, Richard Reeve, Paul C. D. Johnson, Fiona Thorburn, Beatrix von Wissmann, et al. 2019. “Virus–virus Interactions Impact the Population Dynamics of Influenza and the Common Cold.” Proceedings of the National Academy of Sciences of the United States of America 116 (52): 27142–50.
- Pnestad, G. 1982. “INTERFERENCE BETWEEN OUTBREAKS OF RESPIRATORY SYNCYTIAL VIRUS AND INFLUENZA VIRUS INFECTION.” The Lancet. https://doi.org/10.1016/s0140-6736(82)91466-0.
- Polozov, Ivan V., Ludmila Bezrukov, Klaus Gawrisch, and Joshua Zimmerberg. 2008. “Progressive Ordering with Decreasing Temperature of the Phospholipids of Influenza Virus.” Nature Chemical Biology 4 (4): 248–55.
- Shaman, Jeffrey, and Melvin Kohn. 2009. “Absolute Humidity Modulates Influenza Survival, Transmission, and Seasonality.” Proceedings of the National Academy of Sciences of the United States of America 106 (9): 3243–48.
- Shaman, Jeffrey, Virginia Pitzer, Cecile Viboud, Marc Lipsitch, and Bryan Grenfell. 2009. “Absolute Humidity and the Seasonal Onset of Influenza in the Continental US.” PLoS Currents 2 (December): RRN1138.
- Viboud, Cécile, Wladimir J. Alonso, and Lone Simonsen. 2006. “Influenza in Tropical Regions.” PLoS Medicine.
View all COVID-19 updates
Learn about research in the Division of Pulmonary and Critical Care Medicine