Why COVID-19 Is Not like HAPE
The FLARE Four
- The presenting symptoms of COVID-19 and high altitude pulmonary edema (HAPE) are common to many acute respiratory illnesses, and do not constitute a unique link between the two processes
- HAPE is a non-inflammatory process occurring when low atmospheric oxygen pressure triggers excessive hypoxic pulmonary vasoconstriction, resulting in markedly elevated pulmonary arterial pressure and non-cardiogenic pulmonary edema
- The primary treatment of HAPE is descent to lower altitude and supplemental oxygen therapy. This corrects the root cause of HAPE, hypoxic pulmonary vasoconstriction. The resulting edema typically resolves within 6-48 hours
- There is no pathophysiologic or clinical basis for comparing COVID-19 to HAPE. Further, this comparison risks misguided use of HAPE therapies which may harm patients with COVID-19 by worsening ventilation-perfusion mismatch
This piece is courtesy of Dr. Andrew M. Luks from the Division of Pulmonary, Critical Care and Sleep Medicine at the University of Washington.
Subscribe to the latest updates from FLARE Advances in Motion
Many people are saying...because they're blue, maybe they have HAPE.
Amid the Numerous Observations Made Up to This Point in the Pandemic
One idea which has been spread widely via social media is that COVID-19 is not a typical form of ARDS and, instead, is more akin to high altitude pulmonary edema (HAPE) (Solaimanzadeh 2020). Drawing on this purported similarity, some have suggested that medications used in the management of HAPE and other acute altitude illnesses might have a role in the management of COVID-19. Putting aside the obvious fact that the majority of cases of COVID-19 are taking place at elevations far below those at which HAPE develops (> 2400 m), there are multiple reasons why these arguments are incorrect. The entities are, in fact, quite different and efforts to treat patients suffering from COVID-19 with medications used in the management of HAPE will yield no benefit and may lead to unintended adverse consequences.
To lay out this argument, we can first consider the areas of similarity between the two entities that lead to arguments that they are similar. We will then consider important differences in the underlying pathophysiological mechanisms that clearly distinguish the two. Finally, we will examine the problems associated with using HAPE-directed therapies in the management of COVID-19.
What Similarities Do These Entities Share?
There are several similarities between the clinical features of HAPE and COVID-19 which are worth addressing.
They are Both Non-cardiogenic Forms of Pulmonary Edema
Similar to ARDS from other causes, immersion pulmonary edema, negative pressure pulmonary edema, neurogenic pulmonary edema, and reexpansion pulmonary edema, HAPE and COVID-19 are both non-cardiogenic forms of pulmonary edema, meaning that edema develops in the setting of normal left atrial pressure. The common feature of all of these forms of pulmonary edema is that they develop due to an imbalance in Starling forces. As will be discussed in greater detail below, however, the reasons for that imbalance develop for markedly different reasons in HAPE and COVID-19.
Hypoxemia is a Defining Feature of the Clinical Presentation
As documented in several recently published case series, critically ill patients with COVID-19 often present with severe hypoxemia. In the largest case series of ICU patients to date, Grasselli et al. reported a median PaO2/FiO2 of 160 (IQR 114-220) across 1591 Italian patients (Grasselli et al. 2020). Other smaller case series showed a similar median and distribution (Bhatraju et al. 2020; Arentz et al. 2020). All individuals who ascend to high altitude (>2800 m) develop hypoxemia but at any given elevation, those with HAPE will have far worse oxygenation. For example, individuals with HAPE at 4559 m have been reported to have mean PaO2 and SaO2 of 23 + 3 mm Hg of 48 + 8%, respectively, compared to 40 + 5 mm Hg and 78 + 7%, in healthy controls at the same elevation (Bartsch et al. 1987). Similarly, in a study of 8 patients presenting with concurrent HAPE and high altitude cerebral edema (HACE), Hackett el al. reported oxygen saturations ranging between 24% and 74% after evacuation from mountain communities between 2500 and 3000 m down to 1500 m (Hackett et al. 2019).
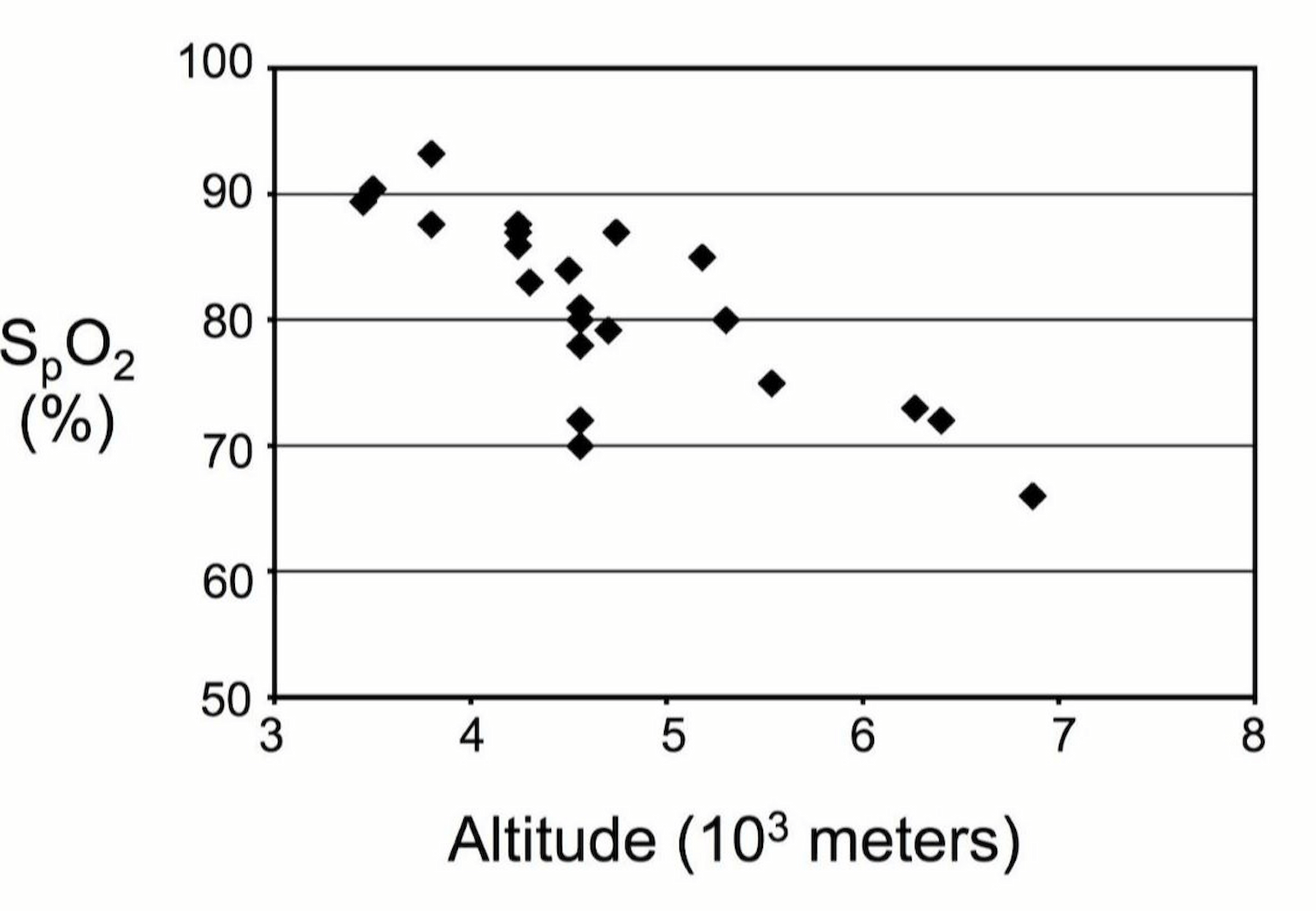
Figure 1
SpO2 measured at various altitudes in healthy individuals (Luks and Swenson 2011).
Dyspnea
In addition to hypoxemia, patients with COVID-19 quite often present with dyspnea, although it has been observed that a subset of patients are noted to be hypoxemic without reports of dyspnea or significant increases in work of breathing, a phenomenon unofficially referred to as “hypoxemia out of proportion to dyspnea.” Dyspnea is also a common presenting symptom in HAPE. In the early stages of that disease, affected individuals note increasing dyspnea on exertion that is out of proportion to what they should experience at that altitude, increased need for rest breaks and prolonged recovery times. As HAPE progresses, dyspnea is present with simple activities, such as walking, or going to the bathroom, and eventually at rest. There is a small subset of HAPE individuals who also do not report significant dyspnea but are noted to have declining performance while exerting themselves or have severe fatigue or poor color when sitting at rest. Rather than being unique to HAPE and COVID-19, this phenomenon can be seen with many forms of hypoxemic respiratory failure. As described in a prior edition of FLARE, dyspnea has a complex relationship to hypoxemia and is mediated by many factors aside from the PaO2 alone.
Radiologic Imaging
Diffuse or patchy bilateral opacities are a common feature of patients presenting with COVID-19 as well as HAPE, although in the latter, there can be considerable asymmetry with opacities often developing initially in the right middle lobe. Bilateral ground glass opacities as well as areas of denser consolidation and air bronchograms have been described in patients with COVID-19 (Dai et al. 2020). There are only limited descriptions of CT findings in HAPE in the literature, as the diagnosis is typically made with plain chest radiography alone. The available reports describe patchy acinar and lobar ground glass opacities as well as denser areas of consolidation (Lindholm et al. 2018) (Figure 2).
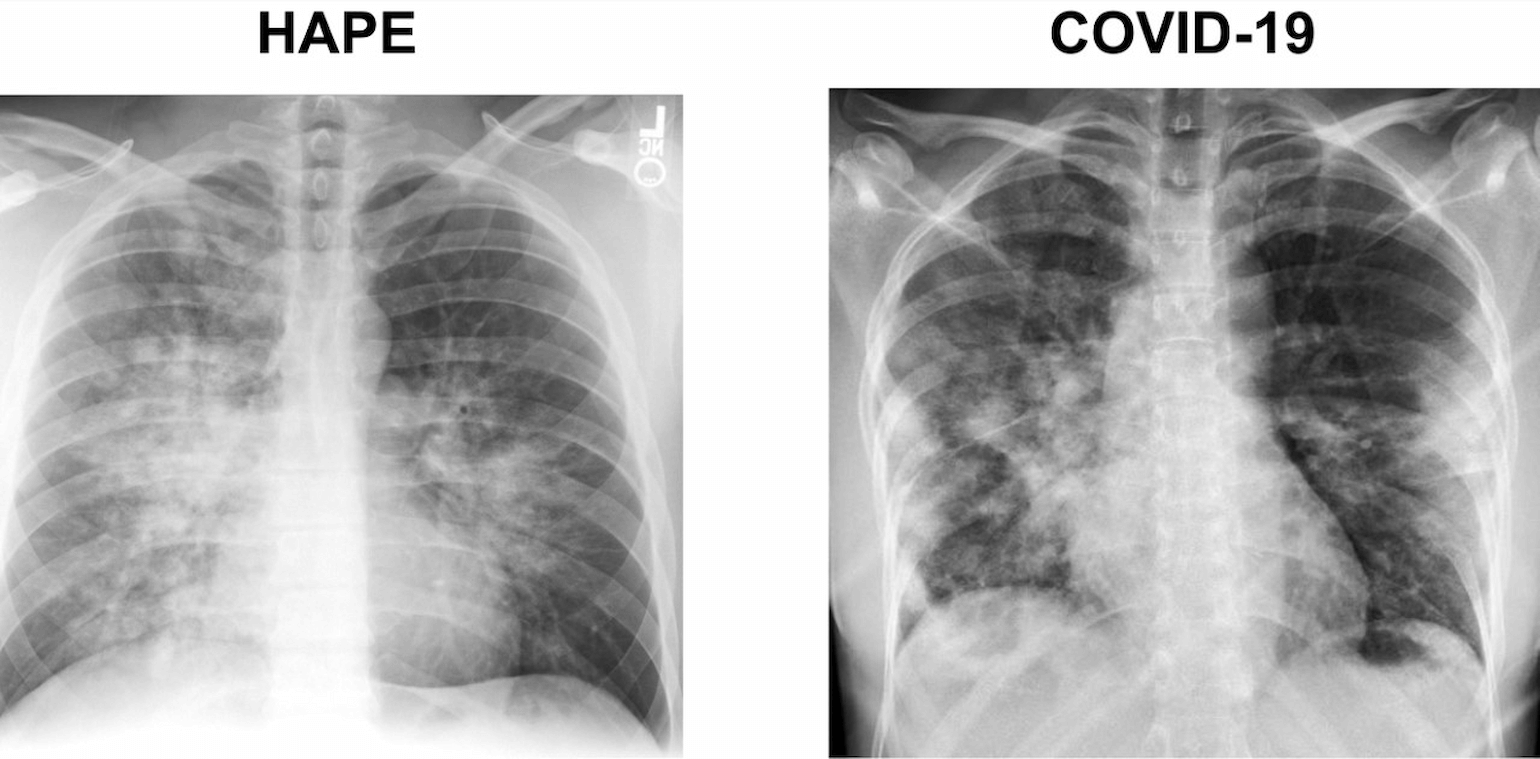
Figure 2
Comparison of CXR and CT scans in HAPE vs COVID-19.
Pulmonary Hypertension
Pulmonary hypertension has long been recognized as a critical feature of HAPE (Hultgren et al. 1964). More recently, Maggiorini et al. measured mean pulmonary artery pressure by right heart catheterization in 16 subjects with HAPE and 14 healthy controls at 4559 m (Maggiorini et al. 2001). They found higher pressures in those with HAPE (37 + 2 vs 26 + 1 mm Hg). Pulmonary artery pressure data has yet to be reported in COVID-19 but there is ample evidence in the ARDS literature of increased pulmonary artery pressure and even acute cor pulmonale (Tomashefski et al. 1983; Mekontso Dessap et al. 2016). A key difference, however, is that in HAPE, increased pulmonary artery pressure, which results from acute heterogenous hypoxic pulmonary vasoconstriction (see below), is the major cause of pulmonary edema, whereas in COVID-19 and other forms of ARDS, pulmonary hypertension is a consequence of the pulmonary edema and various other processes, which are in turn driven by a primary lung pathology.
Decreased Lung Compliance
Reduced respiratory system compliance, similar to values seen in major treatment trials for ARDS (Papazian et al. 2010; Guérin et al. 2013) has been reported in COVID-19 (Bhatraju et al. 2020). However, other anecdotal and published reports suggest that there is considerable heterogeneity and some patients, particularly early in their hospital course, may have only mildly decreased compliance (Gattinoni et al. 2020). Static lung compliance has not been reported in HAPE but indirect evidence from studies utilizing pulmonary function testing suggest this parameter is, in fact, reduced (Clarenbach et al. 2012). The magnitude of reduction is not clear from these studies but the fact that most patients can be treated without the need for invasive mechanical ventilation suggests that decrement in compliance is likely not severe.
Pathophysiologic Differences Between HAPE and COVID-19
While HAPE and COVID-19 are both non-cardiogenic forms of pulmonary edema, and may share some clinical features common to many acute respiratory diseases, there are important differences between them, the most important of which is their underlying pathophysiological mechanisms.
HAPE
High altitude pulmonary edema is the result of excessive hypoxic pulmonary vasoconstriction (HPV). This leads to a marked rise in pulmonary artery pressure and downstream pulmonary capillary pressure. As hydrostatic pressure rises in the capillaries, fluid, protein and red cells leak from the vascular space into the interstitial and alveolar spaces (Swenson and Bärtsch 2012). This often raises the question as to why pulmonary edema is not seen in patients with pulmonary artery hypertension. The key distinction with HAPE is that HPV is heterogeneous; pressure rises throughout the pulmonary circulation, but under the increased driving pressure more flow is directed towards those areas in which HPV does not occur or occurs to a lesser extent. It is here where the leak begins. Importantly, this is a non-inflammatory process. Whereas some early studies demonstrated increased inflammatory markers in the BAL fluid of individuals with HAPE (Schoene et al. 1988) more careful studies, in which BAL was performed early in the clinical course of HAPE, demonstrated that inflammation is not present (Swenson et al. 2002).
COVID-19 and ARDS
In contrast, inflammation and, in particular, the innate immune response, plays a key role in the development of COVID-19 and other forms of ARDS. This response damages the alveolar endothelium and epithelium, increasing capillary permeability and leading to fluid accumulation in the alveolar and interstitial spaces. Alveolar macrophages play a large role in this process by secreting pro-inflammatory cytokines that recruit additional inflammatory cells and activate alveolar epithelial cells and effector T-cells in a manner that sustains the inflammation and promotes ongoing lung injury (Thompson et al. 2017). Together these processes cause loss of surfactant function and alveolar filling and collapse, the net result of which is significant ventilation-perfusion mismatch and hypoxemia.
Treatments Directed at HAPE Will Not Improve COVID-19 and May Make Things Worse
The differences in underlying mechanisms described above highlight a key reason why these diseases should not be viewed as similar entities. In the face of these important differences, it is critical to resist the urge to utilize treatments for HAPE in the management of COVID-19 (aside from supplemental oxygen), since some of the proposed interventions have little physiologic basis but do carry a risk of harm.
The Role of Oxygen
Oxygen, or descent to lower elevation when oxygen is not available, not only corrects the hypoxemia in HAPE but also resolves the underlying pathophysiological problems and pulmonary edema. By raising alveolar PO2, supplemental oxygen decreases HPV which, in turn, decreases pulmonary artery pressure, capillary over-perfusion and the hydrostatic pressure gradient that was driving edema formation. Most individuals experience complete resolution within 6-48 hours depending on the severity of the initial presentation and timeliness of accessing care.
Supplemental oxygen is obviously critical in the management of COVID-19 but its primary role is simply to correct the hypoxemia rather than resolve the underlying disease process. Along with good supportive care and, in many cases long courses of mechanical ventilatory support, supplemental oxygen administration buys the patient time while the lung injury hopefully resolves on its own.
Pulmonary Vasodilators
When oxygen is not available and descent infeasible, the calcium channel blocker nifedipine or the phosphodiesterase-5 inhibitors sildenafil and tadalafil are often used to treat HAPE. Similar to oxygen, they blunt HPV, thereby lowering pulmonary artery pressure and decreasing the hydrostatic pressure gradient causing pulmonary edema. Administration to patients with COVID-19 may lower pulmonary artery pressure and perhaps improve right ventricular function in some patients, but carries a significant risk of worsening oxygenation. By releasing HPV throughout the lung, nifedipine and other systemically delivered pulmonary vasodilators will increase flow to poorly or non-ventilated regions of the lung. This will worsen ventilation-perfusion matching with subsequent deleterious effects on arterial oxygenation. Pulmonary vasodilators like prostacyclin and nitric oxide do have a role in select patients with COVID-19 with the key difference being that they are administered in inhaled form and are only delivered to well-ventilated regions of the lung. This causes selective vasodilation in these areas without affecting HPV in poorly and non-ventilated regions, thereby improving ventilation-perfusion matching.
Acetazolamide
Acetazolamide, which is more typically used in the prevention and treatment of AMS rather than HAPE (Luks et al. 2019) has also been mentioned as a potential therapeutic agent in COVID-19 (Solaimanzadeh 2020). As with nifedipine, the approach lacks a solid physiologic rationale and has the potential to cause harm. One reason invoked for using acetazolamide is that by virtue of its respiratory stimulant properties it can improve ventilation and oxygenation in individuals with blunted ventilatory responses to hypoxemia, the so-called “hypoxemia out of proportion to dyspnea” patients. Acetazolamide may increase ventilation and the alveolar PO2 but in the setting of significant ventilation-perfusion inequality, the small gains in alveolar PO2 will not affect arterial oxygenation to a significant extent, particularly if patients are already on supplemental oxygen. This increase in ventilation may come at the expense of increased respiratory muscle fatigue. In addition, by causing a metabolic acidosis, acetazolamide may worsen dyspnea and work of breathing in those individuals who do, in fact, experience dyspnea in response to hypoxemia. Acetazolamide has also been shown in both animal (Shimoda et al. 2007) and human (Teppema et al. 2007) studies to blunt HPV and, like nifedipine, could contribute to worsening ventilation-perfusion matching. While acetazolamide is not as strong a diuretic as furosemide or bumetanide, intravascular volume depletion may have adverse effects in patients who are hypotensive as a result of the systemic inflammatory response to COVID-19. Finally, depending on the doses administered and the patient’s underlying renal function, acetazolamide may reach serum concentrations sufficient to inhibit red blood cell carbonic anhydrase with subsequent deleterious effects on carbon dioxide transport (Adamson and Swenson 2017).
Conclusion
HAPE and COVID-19 share several features in common including hypoxemia, bilateral opacities on chest imaging, changes in respiratory system compliance, and pulmonary hypertension. These are all non-specific attributes of many acute respiratory disorders however and their presence in both COVID-19 and HAPE in no way implies these entities are at all related to each other. The pathophysiological mechanisms underlying these disorders are fundamentally different from each other, as are the treatment approaches. Attempts to treat COVID-19 with medications typically used in the management of HAPE are likely going to be of no benefit and may even harm the patients. In this era of significant uncertainty about the best ways to treat COVID-19, it is worthwhile to think outside the box and consider alternative approaches. The HAPE box, however, should be closed and stowed away in a cabin up high in the mountains.
References
- Adamson, Rosemary, and Erik R. Swenson. 2017. “Acetazolamide Use in Severe Chronic Obstructive Pulmonary Disease. Pros and Cons.” Annals of the American Thoracic Society 14 (7): 1086–93.
- Arentz, Matt, Eric Yim, Lindy Klaff, Sharukh Lokhandwala, Francis X. Riedo, Maria Chong, and Melissa Lee. 2020. “Characteristics and Outcomes of 21 Critically Ill Patients With COVID-19 in Washington State.” JAMA: The Journal of the American Medical Association. https://doi.org/10.1001/jama.2020.4326.
- Bartsch, P., U. Waber, A. Haeberli, M. Maggiorini, S. Kriemler, O. Oelz, and W. P. Straub. 1987. “Enhanced Fibrin Formation in High-Altitude Pulmonary Edema.” Journal of Applied Physiology 63 (2): 752–57.
- Bhatraju, Pavan K., Bijan J. Ghassemieh, Michelle Nichols, Richard Kim, Keith R. Jerome, Arun K. Nalla, Alexander L. Greninger, et al. 2020. “Covid-19 in Critically Ill Patients in the Seattle Region—case Series.” The New England Journal of Medicine. https://www.nejm.org/doi/full/10.1056/NEJMoa2004500.
- Clarenbach, Christian F., Oliver Senn, Andreas L. Christ, Manuel Fischler, Marco Maggiorini, and Konrad E. Bloch. 2012. “Lung Function and Breathing Pattern in Subjects Developing High Altitude Pulmonary Edema.” PLoS ONE. https://doi.org/10.1371/journal.pone.0041188.
- Dai, Hui, Xin Zhang, Jianguo Xia, Tao Zhang, Yalei Shang, Renjun Huang, Rongrong Liu, et al. 2020. “High-Resolution Chest CT Features and Clinical Characteristics of Patients Infected with COVID-19 in Jiangsu, China.” International Journal of Infectious Diseases: IJID: Official Publication of the International Society for Infectious Diseases, April. https://doi.org/10.1016/j.ijid.2020.04.003.
- Gattinoni, Luciano, Silvia Coppola, Massimo Cressoni, Mattia Busana, and Davide Chiumello. 2020. “Covid-19 Does Not Lead to a ‘Typical’ Acute Respiratory Distress Syndrome.” American Journal of Respiratory and Critical Care Medicine, March. https://doi.org/10.1164/rccm.202003-0817LE.
- Grasselli, Giacomo, Antonio Pesenti, and Maurizio Cecconi. 2020. “Critical Care Utilization for the COVID-19 Outbreak in Lombardy, Italy: Early Experience and Forecast During an Emergency Response.” JAMA: The Journal of the American Medical Association, March. https://doi.org/10.1001/jama.2020.4031.
- Guérin, Claude, Jean Reignier, Jean-Christophe Richard, Pascal Beuret, Arnaud Gacouin, Thierry Boulain, Emmanuelle Mercier, et al. 2013. “Prone Positioning in Severe Acute Respiratory Distress Syndrome.” The New England Journal of Medicine 368 (23): 2159–68.
- Hackett, P. H., P. R. Yarnell, D. A. Weiland, and K. B. Reynard. 2019. “Acute and Evolving MRI of High-Altitude Cerebral Edema: Microbleeds, Edema, and Pathophysiology.” AJNR. American Journal of Neuroradiology 40 (3): 464–69.
- Hultgren, H. N., C. E. Lopez, E. Lundberg, and H. Miller. 1964. “PHYSIOLOGIC STUDIES OF PULMONARY EDEMA AT HIGH ALTITUDE.” Circulation 29 (March): 393–408.
- Lindholm, Peter, Erik R. Swenson, Santiago Martínez-Jiménez, and H. Henry Guo. 2018. “From Ocean Deep to Mountain High: Similar Computed Tomography Findings in Immersion and High-Altitude Pulmonary Edema.” American Journal of Respiratory and Critical Care Medicine 198 (8): 1088–89.
- Luks, Andrew M., Paul S. Auerbach, Luanne Freer, Colin K. Grissom, Linda E. Keyes, Scott E. McIntosh, George W. Rodway, Robert B. Schoene, Ken Zafren, and Peter H. Hackett. 2019. “Wilderness Medical Society Clinical Practice Guidelines for the Prevention and Treatment of Acute Altitude Illness: 2019 Update.” Wilderness & Environmental Medicine. https://doi.org/10.1016/j.wem.2019.04.006.
- Luks, Andrew M., and Erik R. Swenson. 2011. “Pulse Oximetry at High Altitude.” High Altitude Medicine & Biology 12 (2): 109–19.
- Maggiorini, M., C. Mélot, S. Pierre, F. Pfeiffer, I. Greve, C. Sartori, M. Lepori, M. Hauser, U. Scherrer, and R. Naeije. 2001. “High-Altitude Pulmonary Edema Is Initially Caused by an Increase in Capillary Pressure.” Circulation 103 (16): 2078–83.
- Mekontso Dessap, Armand, Florence Boissier, Cyril Charron, Emmanuelle Bégot, Xavier Repessé, Annick Legras, Christian Brun-Buisson, Philippe Vignon, and Antoine Vieillard-Baron. 2016. “Acute Cor Pulmonale during Protective Ventilation for Acute Respiratory Distress Syndrome: Prevalence, Predictors, and Clinical Impact.” Intensive Care Medicine 42 (5): 862–70.
- Papazian, Laurent, Jean-Marie Forel, Arnaud Gacouin, Christine Penot-Ragon, Gilles Perrin, Anderson Loundou, Samir Jaber, et al. 2010. “Neuromuscular Blockers in Early Acute Respiratory Distress Syndrome.” The New England Journal of Medicine 363 (12): 1107–16.
- Schoene, R. B., E. R. Swenson, C. J. Pizzo, P. H. Hackett, R. C. Roach, W. J. Mills Jr, W. R. Henderson Jr, and T. R. Martin. 1988. “The Lung at High Altitude: Bronchoalveolar Lavage in Acute Mountain Sickness and Pulmonary Edema.” Journal of Applied Physiology 64 (6): 2605–13.
- Shimoda, Larissa A., Trevor Luke, J. T. Sylvester, Hui-Wen Shih, Ahamindra Jain, and Erik R. Swenson. 2007. “Inhibition of Hypoxia-Induced Calcium Responses in Pulmonary Arterial Smooth Muscle by Acetazolamide Is Independent of Carbonic Anhydrase Inhibition.” American Journal of Physiology. Lung Cellular and Molecular Physiology 292 (4): L1002–12.
- Solaimanzadeh, Isaac. 2020. “Acetazolamide, Nifedipine and Phosphodiesterase Inhibitors: Rationale for Their Utilization as Adjunctive Countermeasures in the Treatment of Coronavirus Disease 2019 (COVID-19).” Cureus 12 (3): e7343.
- Swenson, Erik R., and Peter Bärtsch. 2012. “High-Altitude Pulmonary Edema.” Comprehensive Physiology 2 (4): 2753–73.
- Swenson, Erik R., Marco Maggiorini, Stephen Mongovin, J. Simon R. Gibbs, Ilona Greve, Heimo Mairbäurl, and Peter Bärtsch. 2002. “Pathogenesis of High-Altitude Pulmonary Edema.” JAMA. https://doi.org/10.1001/jama.287.17.2228.
- Teppema, Luc J., George M. Balanos, Craig D. Steinback, Allison D. Brown, Glen E. Foster, Henry J. Duff, Richard Leigh, and Marc J. Poulin. 2007. “Effects of Acetazolamide on Ventilatory, Cerebrovascular, and Pulmonary Vascular Responses to Hypoxia.” American Journal of Respiratory and Critical Care Medicine 175 (3): 277–81.
- Thompson, B. Taylor, B. Taylor Thompson, Rachel C. Chambers, and Kathleen D. Liu. 2017. “Acute Respiratory Distress Syndrome.” New England Journal of Medicine. https://doi.org/10.1056/nejmra1608077.
- Tomashefski, J. F., Jr, P. Davies, C. Boggis, R. Greene, W. M. Zapol, and L. M. Reid. 1983. “The Pulmonary Vascular Lesions of the Adult Respiratory Distress Syndrome.” The American Journal of Pathology 112 (1): 112–26.
View all COVID-19 updates
Learn about research in the Division of Pulmonary and Critical Care Medicine